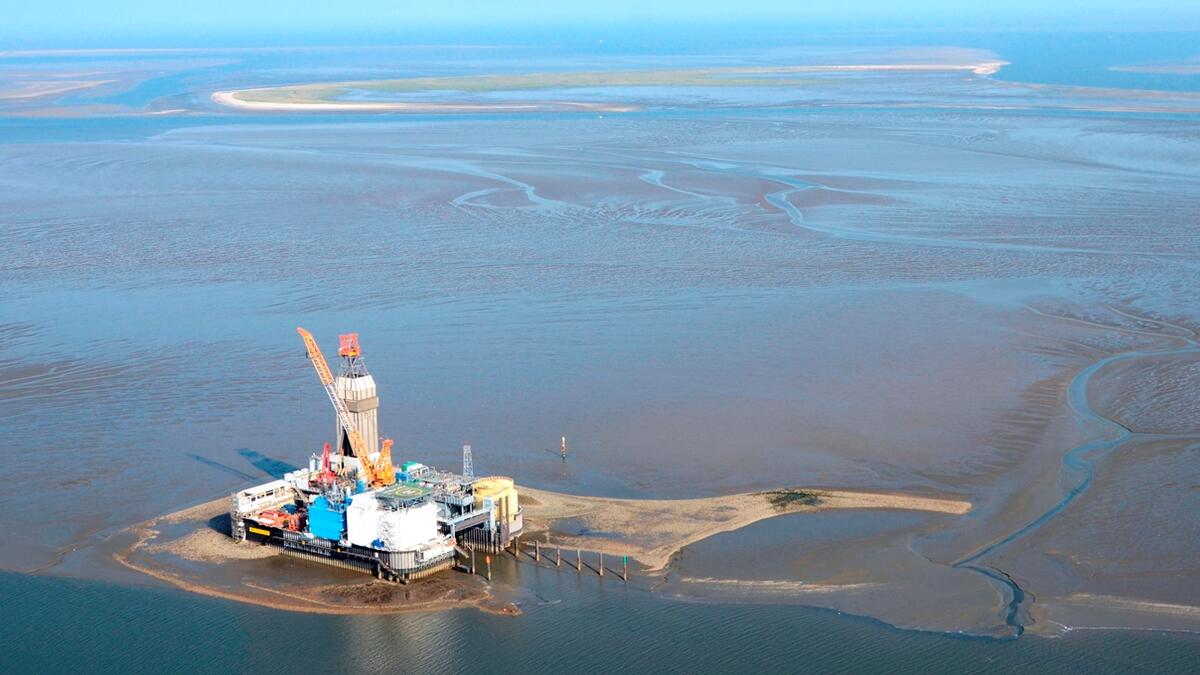
Photo: Media library www.Mittelplate.de. Oil platform Mittelplate.
Energy
S. Christoph, H. Büttger, M. Bauer, J. Baer & G. Nehls
Published 2022
(Version 1.01)
1. Introduction
Day-to-day life in Europe depends on energy, both for private consumption and for the economy. Energy production in Europe is based on fossil, nuclear and renewable energy (ec.europa.eu/eurostat). The burning of fossil fuels releases large amounts of greenhouse gases and is mainly responsible for global warming, which is a major threat to the environment. This is particularly true for the sensitive Wadden Sea area, which will suffer especially from sea level rise. The international community has agreed to keep global warming below 1.5 °C, as set out in the Paris Climate Agreement in 2015. To achieve this goal, greenhouse gas emissions must be brought to zero by mid-century. Therefore, a transition from fossil fuels towards renewable energies is essential. In the three Wadden Sea countries, the Netherlands, Germany and Denmark, wind power from offshore plays an increasingly important role in this energy transition. Wind energy at sea has a better yield due to stronger and more constant winds compared to onshore wind energy. The North Sea is a shallow shelf sea, making it ideal for the construction of wind turbines. Up to now, the three Wadden Sea countries have installed more than 2,000 wind turbines in the North Sea with a total capacity of around 12 gigawatt (GW). By 2030, these numbers will have at least tripled.
The expansion of offshore wind energy utilization can lead to impacts on habitats and species. The Wadden Sea area is largely exempt from wind energy, but impacts may also arise from outside. For grid connection of offshore wind farms and interconnection between European countries, it is necessary to lay and operate sea cables through the Wadden Sea with direct effects for the site. In the future wind energy hubs are planned in the North Sea that produce biofuels such as hydrogen which need to be transported to shore via pipelines (HaskoningDHV Nederland, 2021, see also ens.dk).
Furthermore, the Wadden Sea is used to exploit fossil energy. Crude oil is exploited from one platform in the German Wadden Sea and there are several gas mining projects in the Netherlands that extend under the Wadden Sea. Oil and gas pipelines traverse the Wadden Sea which require monitoring and maintenance.
This report gives an overview about the development of energy production in and adjacent to the Wadden Sea and describes major impacts. The Wadden Sea is a designated World Heritage Site. Conflicts of energy production and transmission with the key values derived from the Outstanding Universal Value of the World Heritage are identified.
This report refers to current political plans (status Oct. 2021) up to the year 2030 with a view to long-term planning up to 2040 and beyond.
2. Status and trends
2.1 Wind Energy
The Wadden Sea Conservation Area is exempt from wind farm development. This has been postulated in the Danish Statutory Order, the German National Park Acts and the Dutch Conservation Area (PKB Area). However, the construction of offshore wind farms in the adjacent sea areas has been rapidly increasing in the past years, and the grid connections to the mainland affect the Wadden Sea area directly. Further, on some of the Wadden Sea islands, wind turbines have been erected and wind farms are widespread over the coastal zone adjacent to the Wadden Sea. The following chapters provide an overview of the most recent developments in the wind energy sector and the long-term governmental planning.
2.1.1. Overview
The European Union aims at 55 % reduction of greenhouse gas emissions until 2030 and being climate neutral until 2050 (ec.europa.eu). To reach these targets, offshore wind energy will play an important role. In November 2020, the European Commission proposed in its EU Offshore Renewable Energy Strategy to increase Europe’s offshore wind energy capacity to at least 60 GW by 2030 and to 300 GW by 2050 (ec.europa.eu). Total wind energy accounted for 16.4 % of electricity demand across the EU and UK in 2020 (windeurope.org). A compilation of climate targets and current numbers of the EU and the Wadden Sea states Germany, Denmark and the Netherlands in particular is given in Table 1.
The Dutch government aims to promote energy conservation and renewable energy. Under its Energy Agreement for Sustainable Growth47, the Dutch government has set a target of 16 % of all energy to be generated from renewable sources by 2023 and a minimum of 27 % in 2030 (www.europarl.europa.eu). Greenhouse gas emissions have to be reduced to 49 % by 2030 and 95 % by 2050 compared to 1990 level (Dutch Climate Act 2019).51 Offshore wind farms with a capacity of 11 GW are planned to be installed until 2030. Current plans call for doubling this Figure in order to reach the EU’s recent 55 % reduction target of greenhouse gas emissions (www.rijksoverheid.nl).
With the revision of the Renewable Energy Sources Act (RES-Act) in 2021, expansion targets of renewable energy in Germany have been raised up to 65 % of the gross electric consumption by 2030 (www.bmwi.de). In 2020, 45.4 % of electric consumption was covered by renewables, more than half of it by wind energy (Umweltbundesamt, 2021). The target of 18 % for renewables in gross final energy consumption in 2020 was achieved.14 By 2030 the share is to be increased to 30 %. Germany aims to reduce greenhouse gas emissions by 65 % in 2030 and 88 % in 2040 (Federal Climate Change Act, KSG)58. Climate neutrality is to be achieved by 2045. The targets for offshore wind energy were raised to 20 GW until 2030 and to 40 GW until 2040 by the latest amendment of the Wind Energy at Sea Act (WindSeeG).8 The new government that came into force in the end of 2021 even envisages an increase to 30 GW by 2030, 40 GW by 2035 and 70 GW by 2045 in its coalition agreement (www.spd.de/koalitionsvertrag2021).
By 2030, Denmark aims to cover 55 % of its total energy demand from renewable sources, with offshore wind energy as the main driver of this development as set out in the Energy Agreement 2018.59 Until then, Denmark plans to reduce its emissions by 70 % compared to 1990 level. Independence from fossil fuels shall be achieved by 2050 (Climate Act 2019).
Wind energy will make up a very large part of the energy mix by then. In 2020, 48 % of Denmark’s electricity supply came from wind power, which is the highest share in Europe (windeurope.org). Figure 1 shows the current state of offshore wind farm developments for the Netherlands, Denmark and Germany.
Table 1. Climate targets and statistics of the EU, the Netherlands, Germany and Denmark (Sources: [1] EEA, 2022; [2] EC, 2021, [3] European Parliament Think Tank, 2021; [4] Umweltbundesamt, 2022; [5] EC, 2022b; [6] EC, 2022a; [7] EU, 2009; [8] BMJ, 2019; [9] KEFM, 2020d; [10] WindEurope, 2021; [11] EC, 2022c; [12] Government of Netherlands, 2022; [13] BMWK, 2022).
Figure 1. Overview of wind farm developments in Denmark, Germany and the Netherlands, status 10.2021. Development areas are reserved for the use of wind energy, but the spatial extent of the wind farm or farms has not yet been determined. Development sites reserve space for a wind farm with fix spatial extent. Dutch offshore plans have changed recently. For detailed information on more designated or planned wind farms, see Figure 2. For more information on each wind farm visit also www.4coffshore.com.
2.1.2 The Netherlands
At the end of 2021, the Netherlands’ existing offshore and nearshore wind farms will have a combined capacity of 2,833 megawatt (MW) (Table2). Gemini is the offshore wind farm closest to the Wadden Sea, located 60 km north off the coast of the Wadden islands Ameland and Schiermonnikoog.
In September 2014, the Minister of Economic Affairs presented a guideline to parliament, outlining how the Government plans to achieve an upgrade of 1,000 MW at that time to 4,500 MW offshore wind capacity by 2023. The road map set out a schedule of tenders offering 700 MW of development each year in the period 2015 – 2019. The Government has decided to use three offshore wind farm zones off the west coast of the Netherlands for the deployment of the 3,500 MW new offshore wind capacity: Borssele, South Holland Coast and North Holland Coast Wind Farm Zone.
To facilitate the tight schedule of this road map, a new system was designed for the deployment of these wind farms. This approach contributes to the efficient use of space, cost reduction and an acceleration of the deployment of offshore wind energy. Under this new system, the Dutch government designates Offshore Wind Farm Zones (see Figure 2). Any locations outside these are not eligible to receive a permit.
The year 2020 was a record year for offshore wind energy in the Netherlands. The installation of all turbines at the Borssele Wind Farm Zone were completed, adding almost 1,500 MW to the grid in the beginning of 2021. South Holland Coast (1,540 MW) and North Holland Coast (759 MW) are the next wind farms to follow. Start of construction will be in 2021 with an expected commissioning in 2022/23.
The Coalition Agreement and the Climate Agreement (2019) set a new target of approximately 11 GW generated by offshore wind energy by 2030. To meet this objective, more wind farm zones will be developed further away from the coast: West Holland Coast, Ijmuiden Ver and Ten Noorden van de Waddeneilanden (Figure 2), which will be set out for tender in the period 2021-2025.
Additional offshore wind farms will be needed by 2030 to meet the EU’s latest (2021) more stringent climate target of 55 % greenhouse gas reduction. Together with expected deficits by fulfilling reduction targets in other sectors there is a need for the development of further 10,7 GW until 2030 and 38-72 GW until 2050 (Aanvullend Ontwerp Programma Nordzee 2022-2027; www.rijksoverheid.nl). To cover this demand the National Water Programme has decided to designate new wind energy areas with space for 27 GW until 2050 (search areas with space for 34 GW see Figure 2).
There are no wind turbines situated directly in the Dutch Wadden Sea conservation area. The currently constructed wind farm Fryslan is located adjacent to the Wadden Sea in the IJsselmeer directly south from the Afsluitdijk. Fryslan will be operational until the end of 2021. With a capacity of 383 MW, it will be the largest wind farm in inland water (www.windparkfryslan.nl/).
Table 2. Operational and authorized North Sea offshore wind farms in the Netherlands (status 2021).
Figure 2. Operational wind farms (red), under construction or designated offshore wind farm zones, commissioning until 2023 (light red), designated offshore wind farm zones, commissioning until 2030 (orange-red stripes), proposed additional wind energy areas (orange), and search areas for development after 2030 (broad yellow stripes, narrow yellow stripes – additional search areas), taken from Aanvullend Ontwerp Programma Nordzee 2022-2027 (www.rijksoverheid.nl).
2.1.3 Germany
For the planning of offshore wind farms, Germany focused on areas with higher water depth and distances to the coast, to avoid negative effects on inhabitants, tourism, and environmentally sensitive coastal areas.
Offshore wind farm development and grid connection in the EEZ are spatially planned in a central model, which was introduced in 2017. Based on the Wind Energy at Sea Act (WindSeeG) the Site Development plan (Flächenentwicklungsplan (FEP)) is the controlling planning instrument. The FEP 2020 plans the areas for offshore wind energy and grid connections together with the Grid Development Plan Electricity Development Plan Electricity (Netzentwicklungsplan (NEP)) in the North and Baltic Sea in the period from 2026 until at least 2030. The designated Wind Farm Areas are pre-surveyed by the competent authority and auctioned in a competitive procedure (BSH, 2020). Offshore wind farms are grouped for collective ‘cluster’ grid connection and gates where the offshore grid connections enter territorial seas are determined. In addition, the Site Development Plan contains standardized technical specifications and planning principles.
Spatial planning within territorial waters, where the Wadden Sea area is situated, is carried out by the Länder (CPSL, 2010), including the routing of grid connection corridors traversing the Wadden Sea.
Today, offshore wind energy has a total installed capacity of 7,750 MW and accounts for 10,9 % of the annual gross electricity consumption in 2020 (Umweltbundesamt, 2021).
After high growth rates of installed offshore wind energy capacity in the years 2014-2019, expansion slowed down with only 219 MW capacity growth in 2020. For 2021 no new offshore wind farms will go into operation. This expansion gap is caused by the system-change from a fixed compensated price to a tendering process in 2017. The plants awarded in the 2017/2018 tender round will go into operation between 2022 and 2025. Seven wind farms will increase the installed capacity to 10.8 GW by 2025. Wind farm areas needed to achieve the raised expansion target of 20 GW in 2030 will be put out to tender from 2021 on and will be connected to the grid from 2026 onwards (Deutsche WindGuard GmbH, 2021).
At this point, 23 windfarm projects are fully commissioned in the North Sea EEZ (see Figure 1, Table 3). Three wind farms, Nordergründe, ENOVA Ems Emden and Riffgat, are located within territorial waters.
Table 3. Operational and currently constructed North Sea offshore wind farms in Germany (Status 2021).
The construction and operation of wind turbines is not allowed in the German Wadden Sea National Parks. Nevertheless, a total number of 3 wind turbines are located on the islands in Lower Saxony (Figure 3), and there are 18 turbines on the North Frisian Islands (Figure 4), but in areas which are not part of the National Parks.
In Schleswig-Holstein, wind energy turbines located in the hinterland are restricted to so-called priority areas for construction and repowering, which are designated in the Regional Plans (www.schleswig-holstein.de). With the revised regional plans of 2020 on the topic of wind energy use, the Schleswig-Holstein state government defined areas to be kept free of wind farms as well as buffer zones, including a coastal strip along the North Sea coast.
Figure 3. Location and number of installed wind turbines (WEA) on islands off the coast of Lower Saxony. Although it cannot be depicted in this overview map properly all turbines are located in areas which are exempt from the National Park. Source: Energieatlas Niedersachsen, status 2021, modified (pers. comm. I. Thelen, Municipality Borkum).
Figure 4. Location and number of installed wind turbines (WEA) on North Frisian Islands. Source: LLUR 12.2020, 3 still existing turbines on Nordstrand were added.
2.1.4 Denmark
The Danish government is responsible for the deployment of offshore wind farms. To provide a basis for a long-term planning, the government has created a Committee for Future Offshore Wind Turbine Locations, which identifies the most suitable areas for offshore wind farms, considering the geographical conditions as well as various interests for the area, such as shipping, mining and fishing. Based on the recommendations of this committee, politicians decide which farms to put out to tender.
Three licences are required to build a government sponsored offshore wind farm in Danish waters — a licence for preliminary site investigations, a licence to install turbines and a licence to exploit wind power for a given period. All three are granted by one authority, the Danish Energy Agency (DEA).
Currently, there are three established offshore wind farms in the Danish North Sea: Horns Rev 1, Horns Rev 2, and Horns Rev 3, which were fully commissioned in 2002, 2010, and 2019, respectively (Table4, Figure 1). The small nearshore test project Nissum Bredning Vind with four turbines and a capacity of 28 MW was built in 2018 in the Thyboron Canal. These wind farms have a combined capacity of 776 MW.
The nearshore wind farms Vesterhav Nord and Vesterhav South (together 350 MW) were granted a permit in December 2020 with an expected commissioning in 2023. The next big offshore wind farm, which will be put out to tender in 2021, is Thor with a capacity of 1,000 MW.
Table 4. Operational and authorized North Sea offshore wind farms in Denmark 2021.
In February 2021, the Danish parliament decided on an offshore wind energy island in the North Sea, 80 km off the coast of Jutland (located in area Ei4, see Figure 1, ens.dk). This energy hub will gather and distribute the energy of hundreds of surrounding turbines to the Danish mainland and to neighbouring countries and shall be commissioned until 2030. The hub will have an initial capacity of 3 GW with the perspective to be expanded to 10 GW in the following years. In times of surplus energy production, the island will produce green fuels such as hydrogen.
The maritime spatial plan (Havplan), is Denmarks planning tool for spatial uses in the waters of both North and Baltic Sea, comprising territorial waters and the Danish EEZ. It is under consultation in the second half of 2021. For spatially relevant uses, such as renewable energies, extraction of raw materials and fossil fuels as well as storage of CO2, corresponding areas are designated as so-called development zones or for specific purposes, such as cable connections for offshore wind farms. These uses are to be restricted to these defined areas, although the spatial dimensions are quite extensive, and it is expected that these areas will not be fully utilised. An area to produce renewable energies (offshore wind power), (Ev8), is planned near both, the Schleswig-Holstein Wadden Sea National Park to the south, and the Vadehavet National Park to the east (Figure 1).
2.2 Gas and oil
Besides wind energy, the North Sea is also a place for the exploitation of fossil fuels. This chapter gives an overview of the current situation of oil and gas production in the Wadden Sea.
2.2.1 The Netherlands
The Netherlands is the largest producer of natural gas in the European Union. The Groningen field is amongst the 10 largest gas fields in the world and there are hundreds of small gas fields in the Netherlands subsurface. Almost every household uses natural gas. Oil reserves however are limited. Of the almost 50 discovered fields about 15 are in production (www.nlog.nl). Today, around 150 oil- and gas platforms exist in the North Sea (Ontwerp Programma Nordzee 2022-2027). There is currently no oil extraction in the Dutch Wadden Sea.
Drilling for gas in the Wadden Sea conservation area nowadays is prohibited and therefore must take place from the shore with directional drilling using deviated wells, tapping into the gas reserves underneath the Wadden Sea. There are currently five active gas fields within the Dutch Wadden Sea Conservation Zone: Nes, Lauwersoog, Moddergat, Blija-Ferwerderadeel and Ameland-Oost (Nederlandse Olie- en Gasportal) (Figure 5). Zuidwal, which was exploited from a gas platform located within the Wadden Sea, recently ceased production. A pipeline transported the natural gas to the mainland in Harlingen (Figure 5, Figure 9). Total gas production in the Dutch Wadden Sea peaked in 2013, with 2554 million m3 of gas extracted (Figure 6). Further gas fields that are currently explored in the Dutch Wadden Sea are Ternaard, Terschelling and Schiermonnikoog (Figure 5). Six other fields are in the vicinity of the Wadden Sea and their ground surface subsidence can also extend into the area: Anjum, Metslawier, Blija-Zuidoost, Vierhuizen, Groningen and Warffum.
The new coalition of the Dutch government had reached an agreement for the next coalition in December 2021, which states that gas extraction in Groningen is to be phased out as soon as possible and any further extractions should not be conducted in the Wadden Sea but could take place in the North Sea (www.iamexpat.nl). The gas extraction at Ternaard should take place according to the permissions, which is being discussed publicly right now (www.dutchnews.nl).
Due to ground surface subsidence and earthquakes in the province of Groningen, the production of the Groningen gas field is currently scaled down and will be stopped by mid-2022 (www.rijksoverheid.nl).
Figure 5. Producing and planned gas fields in the Dutch Wadden Sea 2021. Data source: NL Olie- en Gasportaal.
Figure 6. Gas production in the Dutch Wadden Sea 2008-2020 (in million m3). Data source: NL Olie- en Gasportaal.
2.2.2 Germany
Lower Saxony
Currently, there is no production of natural gas in the Lower Saxony Wadden Sea area. The two mining fields that existed since the 1970’s, Manslagt Z1 and Leybucht Z1, have ceased production in 2007 and 2008, respectively.
Hamburg
According to the Act of the Hamburg Wadden Sea National Park, the exploitation of energy resources in the Hamburg Wadden Sea area is not allowed.
Schleswig Holstein
There is no natural gas exploitation in the Schleswig Holstein Wadden Sea, but Mittelplate A, Germany’s largest oil field, is situated within the southern part of the Schleswig Holstein National Park (Figure 7). The field was discovered in the Dogger sandstone in 1981, where reserves of 75 million tons were confirmed. Later the size of the deposit was adjusted upward to over 100 million tons. The oil is located in pores of the sandstone layers at a depth of between 2,000 to 3,000 m. The Mittelplate facilities went into production in 1987 from an artificial drilling and production island, which was built 7 km off the coast. The 70 x 95-metre island was designed as a liquid-retarding steel-and-concrete basin on the sand flats, protected by 11m-high sheet piling. The reservoir is exploited by 20 wells - some horizontal or deviated - of up to 3,000 m depth, tapping the oil-bearing sandstone layers. According to the National Park Act, the exploration and exploitation of oil is strictly limited to Mittelplate A and prohibited elsewhere.
Figure 7. Oil platform Mittelplate, with Trischen Island, Nationalpark Schleswig-Holstein, in the background. Source: Wintershall DEA
In 2000, a land-based facility at Dieksand was established. From here, 2,000 m to 3,000 m-long extended-reach production wells tapped into the eastern part of the deposit. This integrated offshore and onshore development has contributed to the more rapid exploitation of the oil field. One 7.5 km stainless steel pipeline was laid from Friedrichskoog-Spitze through the mudflats off the coast and on through the Marner Plate to Mittelplate Island. Since 2005, oil is therefore no longer transferred by ship, but has been pumped through the pipeline from Mittelplate to the processing facilities of the Dieksand Land Station, which increased the overall safety of the oil transport. The combustion of accompanying gas, which was used to produce energy, had been ceased in 2020. The platform is now fully supplied with onshore power.
So far, 37 million tons of oil have been produced from the Mittelplate oil field, a further 15 to 20 million tons are estimated as being technically and economically recoverable (wintershalldea.de). While the production rate at the beginning of extraction was around 200,000 tons of oil per year, production increased and peaked in 2003 with 2 million tonnes. After this, production rates began to decline due to the natural depletion of the reservoir and ranged between 1 and 1.5 million tonnes in the past years (Figure 8). Over 2,300 t of oil are now transported ashore daily.
Figure 8. Oil production 2009-2020 at the offshore platform Mittelplate and the mainland facility Dieksand. Source: annual reports BVEG 2009-2020.
2.2.3 Denmark
According to the Statutory Order on the Nature Reserve Wadden Sea, exploitation of gas and oil in the Danish part of the Conservation Area is prohibited.
2.3 Cables and pipelines
For grid connection of offshore wind farms with the mainland and interconnection of North Sea countries, it is often necessary to cross the Wadden Sea. Furthermore, data cables and pipelines for hydrocarbons traverse the Wadden Sea. Figure 9 gives an overview about operational and planned cable and pipeline connections in the Wadden Sea.
Figure 9. Cable and pipeline routes which traverse the Wadden Sea. The export cable from wind farm Horns Rev 1 crosses the Danish Wadden Sea at the northern tip of the property but is missing here. There are also several supply lines (water, electricity) that are connecting the islands with the mainland, which are also missing. Source: Bundesamt für Seeschifffahrt und Hydrographie, EMODnet Human Activities and Cogea srl lfalco@cogea.it, apititto@cogea.it, Rijkswaterstaat Noordzee and TNO (www.nlog.nl), Landesbetrieb für Küstenschutz, Nationalpark und Meeresschutz Schleswig-Holstein, status: 2021.
2.3.1 The Netherlands
Several cable connections cross the Wadden Sea at the Dutch-German border and land in Eemshaven like the export cable of the Gemini wind farm and two interconnectors. These high-voltage DC cables connect the energy markets of the Netherlands with Norway (NorNed) and Denmark (COBRAcable).
Most of the currently designated Wind Farm Zones in the Netherlands are situated off the west coast of the Netherlands. However, “Ten noorden van de Waddeneilanden” is situated adjacent to the Gemini wind farm (Figure 6). A tender is scheduled to open in 2022 (english.rvo.nl/). The export cables would need to cross the Wadden Sea. Further wind farms will be built north of the Wadden Sea islands until 2030 with a capacity of 6 GW (Figure 2) and another 10 GW which will be developed from 2030 onwards, making further cable crossings through the Wadden Sea necessary. Different cable route alternatives are currently under consultation (HaskoningDHV Nederland, 2021). In addition, the possibility of landing the energy from wind farms in the form of hydrogen as chemically bound energy is currently discussed, but it is unlikely that hydrogen production will be realized before 2030 (HaskoningDHV Nederland, 2021).
Furthermore, there are several gas pipelines traversing the Wadden Sea connecting the offshore production sites and the islands of Texel and Vlieland with the mainland (Figure 9).
2.3.2 Germany
The grid connection cables for the wind farms situated in the EEZ are to be laid spatially concentrated and bundled together within territorial waters. In Lower Saxony, three priority cable routes crossing territorial waters, designated by the Lower Saxony State Spatial Planning Programme 2017 (www.ml.niedersachsen.de), an additional routing will be required once their capacity has been exhausted. Cables shall be laid in such a way, that the available space can be used for as many cable systems as possible, with the transmission power of 900 MW per system at least. Transmission power will be raised to 2 GW in the future from 2030 onwards (BSH, 2020).
The majority of energy connections currently run between the islands of Lower Saxony to reach the mainland (Figure 9). About a dozen offshore grid connections are approved to cross Lower Saxon territorial waters and the Wadden Sea.
The expansion of offshore wind energy to 20 GW by 2030 will make further cable connections necessary. Eight new connections will go into operation in Lower Saxony and one in Schleswig-Holstein by 2030 (NEP, 2019).
In Schleswig-Holstein three grid connections connect offshore wind farms in the EEZ north of the island Heligoland and east of the island Sylt with the mainland bundled via the so-called Büsum cable corridor. Furthermore, Nord.Link, a 525kV HVDC grid connection between Norway and Germany is also traversing the National Park in parallel with these three 380 kV grid connections systems. The high-voltage DC link will enable the exchange of 1,400 MW of renewable energy-wind power from Germany and hydropower from Norway.
There are also some pipelines crossing the German Wadden Sea interconnecting the gas markets from North Sea riparian countries (Figure 9). The oil platform Mittelplate transports crude oil to shore via pipeline as well.
2.3.3 Denmark
The COBRAcable, which connects the Netherlands and Denmark, crosses the Wadden Sea for the second time in Denmark and lands at the mainland in Endrup. The export cable of wind farm Horns Rev 1 traverses the Wadden Sea at the northern tip of the property. No further cable or pipeline connections are currently planned in the Danish Wadden Sea.
3. Assessment
3.1 Overview of the policy and regulation framework
There are numerous international and national guidelines and regulations for the promotion, regulation, and spatial planning of renewable energy (designation of sites for offshore wind farms, construction of wind turbines and grid connections) and for the exploitation of oil and gas in force. These are applied in the exclusive economic zones, the territorial waters or in the Wadden Sea property of the Wadden Sea riparian states (Table5, Table6). The policies are summarised below.
On an international level, OSPAR, which aims to protect the marine environment of the North-East Atlantic, provided a guidance on the identification and consideration of some of the issues associated with determining the environmental effects of offshore wind farm developments in 2003 already.
3.1.1 The Common Policy
There are no offshore wind farms or wind turbines within the Wadden Sea World Heritage property. The Wadden Sea Plan (2010) as the main management document within the framework of the TWSC states the common policy that no wind turbines shall be erected in the Nature Conservation Area. This is also part of the Danish legal ordinance, the German national park laws, and the Dutch nature reserve (PKB area). Furthermore, the Wadden Sea Plan (2010) includes to allow wind turbines outside the Conservation Area only if important ecological and landscape values are not negatively affected. However, the construction of wind farms in the adjacent sea areas has been rapidly growing in the past years. The associated grid connections of offshore wind farms outside the property with the onshore power grids traverse the Wadden Sea World Heritage property as high voltage submarine cables in several corridors. In this regard, the three countries agreed to “concentrate cable crossings through the Wadden Sea within a minimum of cable corridors and a minimum of cables using the best available techniques, e.g., cables with highest capacity available, and to communicate regularly on this item in order to use synergies” (CWSS, 2010). Regarding the production of oil and gas the states have agreed that no new exploration or exploitation installations within the boundaries of the World Heritage Site will be permitted (CWSS, 2010).
3.1.2 National Policies
At the national level, the three riparian states of the Wadden Sea have developed different policies to promote and control wind energy development adjacent to the Wadden Sea (offshore and on the mainland) as well as policies, which regulate/prohibit oil and gas exploitation and wind farms within the Wadden Sea (Table 5, Table 6).
Spatial planning is one of the key instruments to balance competing spatial interests. Bearing in mind the impacts of wind turbines and power cables on the environment, especially on nature and human health, it has widely been recognized, that the construction and operation of wind turbines and high voltage power cables shall be restricted to defined areas.
Table 5. International and national legal framework relevant for wind energy (constructions and grid connections) in or adjacent to the Wadden Sea (2021) (for sources indicated by the footnotes, please see Annex).
Table 6. Exploitation of oil and gas in the Wadden Sea Countries and relevant international and national policies (2021).
3.2 Impact factors and effects
The effects of the construction and operation of renewable or fossil energy are related to various impact factors that are construction-related, operational, or plant-related (Table7). In addition, the above-mentioned or other impact factors may become relevant during the dismantling of facilities; these are not considered here. The following description of the impact factors refers to the current activities related to energy production as compiled in chapter 2. The description delivers a broad overview, project and site- specific impacts cannot be considered so that this compilation cannot make a comprehensive or universally valid description of the impacts, but it provides a good overview.
Table 7. Overview about possible impacts of renewable and fossil energy infrastructure, which might affect the Wadden Sea area during the construction and operation phase and plant-related (indicated with X). Note: * Impact factor "light" is considered within "collisions".
Most offshore wind farms are located far offshore (>10 km away from the border to the Wadden Sea), so that it can be excluded that direct impacts from those wind farms arise for the Wadden Sea area. But there are some exceptions, like the wind farms Nordergründe or Riffgat, which are located 600 m, respectively ca. 4 km from the border of the National Park. In the Netherlands, the near shore wind park Fryslan is situated directly adjacent to the Wadden Sea in the IJsselmeer but is separated by a dam. Currently, Horns Rev 1 is the closest wind park in Denmark located ca. 8 km west of the Danish Wadden Sea. Recent plans envisage an area (Ev8), which is located directly north of the Schleswig-Holstein Wadden Sea and 8.5 km west of the Danish Wadden Sea.
Impact factors are described for the foreseen development of energy activities within the next six years.
3.2.1 Underwater noise
Currently almost all offshore wind energy plants are constructed with steel foundations. These foundations are driven into the sea floor with hydraulic hammers and the help of an installation vessel. Pile driving creates strong underwater noise emissions, which can lead to hearing traumata of marine mammals with temporary or permanent hearing loss at close distances. Especially Harbour porpoises (Phocoena phocoena) are affected as they occur in all offshore wind farm areas of the North Sea. In the Wadden Sea Countries, the use of noise abatement measures is mandatory (Energistyrelsen, 2016; Juretzek et al., 2021). In Germany, the maximum sound exposure level (SEL) must not exceed 160 dB re 1µPa²s in 750 m distance to the construction site (Müller & Zerbs, 2011). This measure aims to ensure that no harming of marine mammals occurs. To meet this requirement every project needs a noise mitigation concept. Noise mitigation can be achieved with different measures like air-bubble curtains, hydro sound dampers, cofferdam or cladding tube (Koschinski & Lüdemann, 2013).
Furthermore, it has to be ensured, that marine mammals leave the close range. Therefore, several acoustic harassment devices are used to scare marine mammals in the vicinity, so-called pinger, seal scarer or Fauna Guard. Those devices emit deterring sounds and are used prior to pile driving in all three countries. Studies have shown that in general those measures are successful and marine mammals leave the area but acoustic harassment also adds up to the total noise stress and devalues habitat for marine mammals (Brandt et al., 2016; Rose et al., 2019).
Alternative foundation techniques are currently under development. The Vibro Pile Driving technique utilizes vibratory hammers which transfer vibration to the monopile. This leads to liquefaction of the surrounding sediment and the monopile sinks into the underground by its own weight. Wind farm “Kaskasi”, which is planned to be built by 2022 north of Helgoland, will use Vibro Pile Driving (www.offshorewind.biz). Such projects can reduce the impact of underwater noise considerably.
For the Wadden Sea, underwater noise related to construction of wind farms is only relevant for locations within several km around to the World Heritage Site, like Nordergründe and Riffgat wind farm. No further wind farms are currently planned in the vicinity of the Wadden Sea. Therefore, there will be no underwater noise related to the construction of wind farms affecting the Wadden Sea area in the near future. Should concrete plans for the Danish wind energy area Ev8 come up, the Wadden Sea could be partly affected by underwater noise. Marine mammals, which might migrate between the Wadden Sea and the open sea, may be affected by piling noise in offshore areas.
Offshore wind farms emit low-frequency operational underwater noise, which lies below the best hearing range of porpoises. Operational noise can be perceived only for several 100 m (Madsen et al., 2006). Therefore, operational noise is not relevant to the Wadden Sea.
Ship traffic due to construction, monitoring or maintenance of wind farms and oil or gas facilities also cause underwater noise, which is considered under the impact of “scaring”.
3.2.2 Reef effect
With the erection of wind farms and oil or gas facilities in the North Sea artificial hard substrate is introduced into an environment where it is naturally scarce. Within a short time, all kinds of submerged hard substrates are overgrown by sessile organisms. Compared to the former habitat, biomass increases strongly. The surrounding seafloor is affected by nutrient enrichment (reviewed in DEGRAER et al. 2020). Other species, like hard substrate-associated fish, can get attracted by an increased food availability (Reubens et al., 2013) with further effects on higher trophic levels like birds and mammals (Russell et al., 2014). Wind farm areas are excluded from fishing. This may lead to a refugium effect for fish, shellfish and commercially used crustaceans with a possible increase in stock size (Ashley et al., 2014; Stenberg et al., 2015; Gimpel et al., 2020).
Monopiles of wind turbines, like other artificial hard substrate, are often colonized by non-indigenous species (Adams et al., 2014; De Mesel et al., 2015). The vast majority of these species have planktic larvae which can be transported over many kilometers with the currents. Wind farms therefore may serve as stepping stones for non-indigenous species (Adams et al., 2014; De Mesel et al., 2015). With the expansion of wind energy, artificial hard substrate will increase in the North Sea over the next decades. However, it is uncertain if the presence of so many wind farms in the North Sea significantly increases the risk of the introduction and settlement of non-indigenous species into the Wadden Sea.
3.2.3 Physical disturbance of the seafloor
The laying of cables through the Wadden Sea leads to physical disturbance of the sea floor. Depending on the applied method the impacted area and the intensity of impacts are very different. The open construction method with moulding cutter and subsequent refilling of the trench with an excavator is a method with relatively high demand of space and a major intervention into the sediment structure. A cable trench of 2.5 m is formed where the sediment layers are deeply mixed up (Wolters, 2012). The surrounding area is impacted by sediment deposition. The use of an excavator to refill the trench in the intertidal leads to sediment consolidation on a working strip of around 15 m (Wolters, 2012). At the cable route, the sediment structure is impaired for several years (Backer et al., 2013).
All jetting systems leave an open trench on the seafloor with down-sliding slopes (see also Figure 17). Mobilized sand particles deposit in or in the vicinity of the trench and lead to a cover of the adjacent seafloor. Smaller particles can be carried over long distances and disperse wide-ranging depending on strength of currents. Jetting sleds further impact the sediment structure by producing skid tracks (Wolters, 2012). It was observed that after cable laying with a vertical injector the trench was closed and morphologically recovered after some weeks (GFN 2012; cited in WOLTERS 2012).
Vibrating tools (vibration cable plough as track vehicle or attached to a swimming unit, Figure 10 and Figure 11) have the advantage that the sediment is just cut and pushed apart and not deeply mixed in the cable trench. Chain tracks of the track vehicle cause sediment consolidation (Wolters, 2012).
BACKER et al. (2013) compared different cable laying methods and found that cable laying with a moulding cutter and excavators had a 10-fold demand of space compared to the mixed use of vibrating cable plough and vertical injector. Even smaller was the demand of space with a vibrating plough attached to a pontoon. This method has therefore been applied in the tidal waters in Lower Saxony and Schleswig-Holstein to mitigate the impacts as far as possible.
Further impacts on the sediment arise from mooring anchors and anchoring ropes. At intersection buildings hard substrate is introduced into a soft sediment environment.
For detailed information about laying techniques, see Annex.
Figure 10. The vibration cable plough is a laying tool utilized for tidal flats (Source: Bohlen & Doyen).
Figure 11. Cable laying with vibrating plogh attached to a barge. It can operate at the lower intertidal and subtidal (Source: Bohlen & Doyen).
In the direct area of the cable trench the mortality of macrobenthic organisms is probably high. Depending on the method, the demand of space and the temporal extend of structural disturbance of sediments can be minimised. How severe and long-lasting the disturbance is, also strongly depends on the habitats affected. To carefully evaluate different route options can therefore make a substantial difference for the ecological impact (as an example see www.waddenmozaiek.nl).
The oil platform Mittelplate was connected to the mainland with a pipeline in 2005. Installation of the pipeline was conducted with horizontal directional drilling (HDD) in 20 m depth. Six prefabricated pipeline sections were connected with building pits in the tidal flats. Thus, the pipeline underpasses the salt marshes and tidal flats with relatively little disturbance of the seafloor.
3.2.4 Sealing /habitat loss
Through the construction of wind farms and oil or gas plants, areas are built over and sealed in the long term or are not available as habitat, at least for a limited period of time. The impact refers to a temporary or complete habitat loss, which occurs e.g. as a result of overbuilding by the wind turbines themselves, the foundations or scour protection. The temporary loss of ecosystem function can occur on the cable trench or as a result of sedimentation and is considered in chapters 3.2.3 and 3.2.5.
3.2.5 Turbidity and Sedimentation
All cable laying methods conducted under water will lead to turbidity plumes of different strengths. Suspended sediments may deposit in areas with low current velocity. It can be assumed that the vibrating tools have no or only a small impact on turbidity whereas jetting systems and moulding cutter mobilize huge amounts of sediment per cable kilometre.
Turbidity can be a threat to water organisms, as it impairs the function of gills and filter-feeding organs (Essink, 1999). Especially juvenile stages of fish and eggs are sensitive (Westerberg et al., 1996). Turbidity also can impair the visual sense of predators and reduce the photosynthetic active radiation for plants. The Wadden Sea is an intertidal water and naturally very rich in suspended matter. Organisms in the ecosystem are adapted to high loads of suspended matter. In addition to the method used, the duration and amount of sediment (turbidity and deposition) are decisive factors that influence the biotic components. Effects can therefore take different lengths of time and regeneration does not necessarily lead to the same species inventory as before (e.g., due to altered grain size composition).
3.2.6 Heat dissipation
High voltage power cables produce dissipative heat, which is transferred to the surrounding medium. Due to safety reasons, sea cables usually are buried in the sediment. To prevent changes in geophysical traits or the benthic ecosystem, in Germany the 2 K-criterion was introduced. It stipulates that the seafloor shall not be heated more than 2 K (2 °C) in 30 cm depth in coastal areas, respectively 20 cm depth in offshore areas (BfS, 2005). Therefore, sea cables are buried in at least 1.5 m depth, in special areas like shipping navigation areas in more than 3 m depth. In the Netherlands sea cables have to be buried in at least 1 m depth (Waterbesluit Artikel 6.16j). High-voltage cables that cross the Danish Wadden Sea are buried as well (pers. comm. Energinet, J. Havsager).
Temperature rise in the surrounding sediment of cables and at the seafloor is dependent on the cable type (AC or DC), transmission rate, sediment properties and ambient temperature. Theoretical models predict, that in German coastal waters and the EEZ the 2 K-Criterion can be met with a cable burial depth of 1 m (reviewed in MEIßNER & SORDYL 2006). In the close vicinity to the cable temperature rise can exceed 30 K. High temperature can alter the sediment chemistry, like changes in redox, O2, sulphides profiles, nutrient profiles and increase in biological and bacterial activity (PROKOP 2006, cited in IFAÖ 2006). Biogeochemical processes that are initiated in deeper sediment layers are in exchange with the surface by pore water contact. Oxygen depletion and formation of “black spots” in the Wadden Sea might be facilitated along the cable routes (Meißner & Sordyl, 2006).
In situ, studies about ecological effects of temperature rise at power cables are missing. Negative impacts on the benthic species diversity were found for habitats affected by cooling water, e.g., from power plants (reviewed in MEIßNER & SORDYL 2006). Though, a direct transfer of these findings to temperature rise at power cables is not appropriate. It is suspected, that warming at power cables can cause changes in physiology, reproduction or mortality of certain benthic species and to a small-scale community shifts towards more thermophilic species along the cable route (OSPAR Commission, 2012; Taormina et al., 2018).
Transmission capacity will be raised from 900 MW towards 2 GW. Increasing the conductor diameter will ensure that heat transmission will remain within the set boundaries (www.bmwi.de). Otherwise, the depth of cable laying is a relevant factor to be considered.
Heat dissipation is also generated by the pipeline connecting oil platform Mittelplate to the mainland. Currently, it is heated up to 62°C to fluidize the crude oil. As the pipeline is layed in 20 m depth, it was excluded that the surface of the seafloor is impacted by temperature rise. A temperature monitoring is obligatory when injection temperature exceeds 70 °C (pers. comm. National Park Authority SH, J. Schrader).
3.2.7 Electromagnetic fields
Undersea cables, which transfer energy over long distances usually use high-voltage direct current (HVDC). Static electromagnetic fields occur as a by-product. The electric compound is almost entirely shielded by the earth and the cable material. The kind of magnetic field and the level of the magnetic flux density correspond to those of the geomagnetic field (www.emf-portal.org).
Three-phase alternating current is normally used for short range power transmission (i.e., interconnection of wind turbines in a wind farm) and was also used for the wind farms located within the 12 sm zone Nordergründe and Riffgat. For long distances, alternating current is not applied because of high energy loss. Alternating currents create alternating electromagnetic fields. In a three-phase power cable the currents are offset in time by one third of the oscillation cycle. As a result, electromagnetic fields cancel each other out to a large extent.
As all sea cables are buried it is assumed, that remaining electromagnetic fields at the sediment surface are weak. Some electrosensitive organisms, like sharks, rays, eels, lampreys and some invertebrates, can sense the fields in the vicinity of the power cables. Possible effects are assumed to be minor and limited to behavioral changes (BfS, 2005; Meißner & Sordyl, 2006). However, electromagnetic fields permanently occur during operation of cables and the number of cables will increase in the future.
3.2.8 Spills
The only place where crude oil is exploited in the Wadden Sea is the oil platform Mittelplate off the coast of Schleswig-Holstein. The artificial island of Mittelplate was constructed under high safety requirements. It is a leak-proof concrete-and-steel basin. A pipeline has been installed 20 m below the seafloor and transports the crude oil to facilities at the mainland. This replaces 2,000 ship movements per year, which mitigates the risk of oil spills. Comprehensive safety regulations which are controlled regularly by the competent authority (State Office for Mining, Energy and Geology Lower Saxony, LBEG) contribute to preventing environmental damage to the birds or the mudflats and tidal waters. Every incident whatsoever occurs has to be reported to the LBEG. There is a reporting schedule acc. to offshore-directive in place which includes also the Schleswig-Holstein Agency for coastal protection, national parks and marine protection (LKN) with the units of responses to accidental pollution and the National Park Authority amongst the relevant authorities. Until now, no oil accidents have occurred, but despite all safety measures a residual risk of accidents cannot be excluded. The oil platform is located close to the major moulting area of shelducks with more than 150,000 individuals (Kempf, 2020). Monitoring of facilities and pipeline routes is important and to our knowledge carried out to prevent oil or gas leakage.
Increased shipping activity due to construction and maintenance of all kinds of activities related to energy production increases the risk of accidents and oil spills. Obstacles like wind turbines can further increase the risk of collision with ships. Oil spills would be detrimental to seabirds, benthic, pelagic and coastal ecosystems as well as for tourism and fishery. When the cargo-ship “Pallas” wrecked off the coast of Amrum in 1998 spilled oil caused the death of 16,000 seabirds (www.landtag.nrw.de). The issue of constant discharge or leakage of oil from ships and facilities is considered under the impact “pollution”.
3.2.9 Ground surface subsidence
Gas exploitation, together with other human subsurface activities (e.g. salt mining), can affect the ground surface level in the Wadden Sea. In the Netherlands, gas is extracted from sandstone reservoirs. When the pressure of the reservoir drops it can be compressed by the overburden load, resulting in ground surface subsidence (Fokker et al., 2018).
There are more than a hundred producing mining projects in the Netherlands, some of which have resulted in ground surface subsidence. The big gas fields underneath the sea floor give rise to the issue of subsidence, especially in sensitive areas like the Wadden Sea, as its existence depends on the ability to adjust to changing sea levels. However, modelling the rate of subsistence in a dynamic environment like the Wadden Sea is very complex, taking into account predicted sea-level rise and sediment displacement. Onshore, continuous GPS stations are installed near subsidence bowl centres, to monitor subsidence rates. They serve as an early warning system. In the Wadden Sea, special buried benchmarks have been installed on which GPS antenna can be mounted temporarily during measurement campaigns.
With a partial extension of its field underneath the Wadden Sea, the Ameland gas field, situated underneath the island of Ameland, is one of the oldest and has been producing since 1986. The production has caused up to 35 cm of subsidence in 2018 and the prognosis for 2050 indicates a maximum subsidence of 42 cm in the area underneath the island (NAM B.V., 2020). Since 1990 the Dutch coastline has been maintained by sand nourishment and therefore subsidence caused by the depletion of gas fields in the coastal zone are compensated by sand suppletions (Schoeman, 2006). Due to the sensitive nature of gas fields in tidal areas, gas fields such as Ameland, Nes, Moddergat, Lauwersoog, and Vierhuizen are being exploited within the so-called ‘effective subsidence capacity’ (ESC). The ESC is the maximum human-induced subsidence, which does not cause significant ecological impact. It is determined by the difference between the maximum sedimentation rate, which a tidal basin can maintain, and the rate of relative sea-level rise (sea-level rise + land subsidence). Gas production is adjusted or halted, an approach known as ‘hand on tap’, if there are signs that the subsidence capacity and six years average expectation values of ESC rates will be exceeded now or in the future. So far, this situation has not occurred. For more details on subsidence management and modelling in the Wadden Sea see also DE WAAL et al. (2012); DE WAAL & SCHOUTEN (2020).
The prediction of ground surface subsidence as well as sea level rise is complex and full of uncertainties.
Models by VAN DER SPEK (2018) predict that, depending on scenario, in the future sea level rise may exceed sedimentation rates, leading to an onset of drowning of tidal basins. Man-made surface subsidence can have a contribution to a possible drowning of intertidal flats.
3.2.10 Collisions
Flying birds have a risk of collision with high vertical structures, like wind turbines, gas and oil platforms and ships. In the offshore environment, this is especially true for nighttime migration under poor visibility (Avery et al., 1977; Aumüller et al., 2011). Effects of obstruction lighting at wind turbines and gas flares can affect birds; they become attracted and collide, or they start circling and get exhausted (die by fatigue) or they get disoriented as certain wavelength can interfere with their navigational abilities (Evans Ogden, 1996; Gehring et al., 2009; Van Doren et al., 2017). Most offshore wind farms are in large distances to the shore. Nevertheless, some breeding birds and species, which stopover in the Wadden Sea during migration, forage in offshore areas or encounter wind turbines on their migration route (Garthe et al., 2016; Gyimesi et al., 2018). Some near shore wind farms are located adjacent to the Wadden Sea and may cause impact on migrating Wadden Sea birds or birds which move back and forth between feeding and roosting sites.
Little is known on fatality numbers at offshore wind farms, as carcass searches are not possible and technical systems to register collisions are not yet available. In case future studies would reveal significant collision rates, mitigation measures might be implemented to reduce the collision risk, such as shutdown (on demand or according to preset schedules) of wind turbines during mass migration events during unfavorable weather conditions (Welcker & Vilela 2019). Demand-controlled nighttime obstruction lighting is obligatory for all wind turbines erected after July 2020 in Germany (§ 9 (8) EEG) which is expected to reduce the attraction of birds to the wind farms at night. The red blinking lights regarding air safety are then switched on only when an airplane approaches, leading to a reduction of light emissions by 95 % (www.dark-sky.com).
Coastal wind farms do not have a generally elevated collision risk (Grünkorn et al., 2009), though some examples (Everaert & Stienen, 2007; Everaert, 2014; Brenninkmeijer & Klop, 2015) point out that this is the case at least for some exposed locations. However, as bird abundance in the coastal zone along the Wadden Sea is usually higher than at most inland sites and there are often interactions with roosting, breeding, and feeding areas of the Wadden Sea, a good spatial planning is essential to keep the collision risk for birds at a low level.
Oil and gas platforms are large and high constructions, which need to be lighted due to safety reasons, pose a risk to birds (see above). Studies showed, that light color can have an impact on the strength of attraction, though studies showed contradictory results (Evans et al., 2007; Van De Laar, 2007; Poot et al., 2008; Hill et al., 2014). Regarding the German oil platform Mittelplate a new light concept introduced in 2011 helped to considerably reduce light emissions (see Figure 12 and Mattfeld et al., 2012).
Figure 12. Oil platform Mittelplate at night before (left) and after (right) modification of the light concept (Source: Wintershall DEA).
3.2.11 Scaring
The behavioral responses of sea birds to wind farms and other vertical structures are species-specific and vary from attraction (cormorants, gulls, red-breasted mergansers) to strong avoidance (great crested grebe, divers, northern gannet) (Dierschke et al., 2016). Divers (Gavia spp.) are the most sensitive species with an avoidance distance of more than 10 km to wind farms (Mendel et al., 2019; Vilela et al., 2020). Moulting shelducks show a minimum avoidance distance of 1 km around the oil platform Mittelplate (Kempf, 2020). Such stationary constructions can therefore cause a habitat loss for several species.
All kinds of energy production increase the ship and air traffic in the Wadden Sea due to construction, maintenance and monitoring of facilities and cable/pipeline connections. Ships emit underwater noise. Dependent on size, engine and velocity the noise can lead to behavioral responses, acoustic interference, harming of the hearing sense and stress of marine mammals (Erbe et al., 2019).
Shipping and air traffic can also scare birds and cause flight reactions. Depending on species reaction to ships can reach from attraction (sea gulls, terns) to avoidance distances of up to 2 km (divers and common scoters) (Bellebaum et al., 2006; Schwemmer et al., 2011; Fliessbach et al., 2019). Moulting birds are especially sensitive against disturbances (Thiel et al., 1992; Dehnhard et al., 2020). Channeled ship traffic can lead to habituation in some species and can reduce the impact on foraging, resting or moulting species (Schwemmer et al., 2011). Maintenance ship traffic from the oil platform Mittelplate can be particularly disturbing to shelducks, as the area is an important moulting area (Kempf, 2020). An existing agreement to bypass major moulting areas for the moulting time could help avoid disturbances.
3.2.12 Pollution
Pollution, comprising marine litter, eutrophication, oil pollution and chemical contamination, is a significant issue in the Wadden Sea (see QSR section on Pollution). For energy production pollution is relevant due to leakage or discharge of hazardous substances from ships, oil and gas platforms as well as from wind turbines.
Oil spillage in the Wadden Sea can be mainly attributed to discharges from ships (Schulz et al., 2017, see thematic report on Oil pollution and seabirds). Oiling is a major threat to sea birds, particularly for offshore species like Common Guillemots. The proportion of oiled birds significantly decreased over the last decades as revealed by the trilateral beached bird survey (Schulz et al., 2017; Camphuysen, 2019), which was related to an effective international legislation and implemented measures to reduce oil pollution (Lagring et al., 2012).
Regarding wind turbines, corrosion protection systems are a source for hazardous substances. These systems are essential to maintain the structural integrity of all submerged structures. Corrosion protection can be achieved with different techniques, which can also be combined (Kirchgeorg et al., 2018): Galvanic “sacrificial” anodes release high amounts of metals, like aluminum, zinc and indium, in the order of several kg (monopiles) to tons (platforms) per year into the environment. Impressed current cathodic protection (ICCP) systems deliver direct current to the steel surface of submerged structures by using chemically inert anodes and have very low emissions of metals. Coatings of submerged structures can serve as additional corrosion protection. Organic compounds, e.g., bisphenols and many others, can be released from coatings due to leaching and weathering process. Despite some knowledge gaps, KIRCHGEORG et al. (2018) consider environmental risks from corrosion systems currently to be low. The massive expansion of offshore wind energy in the future will lead to a need for research on this topic.
Cable laying can cause resuspension of formerly particle-bound contaminants and nutrients in the water column when sediments are moved. Depending on method (see chapter 3.2.5 and Annex) cable laying mobilizes huge amounts of sediment. The impact of resuspension of contaminants and nutrients is not easy to assess in a predominantly dynamic system. In areas where the pollutant load is high, e.g. harbours, an effect on the environment cannot be excluded, although water currents will lead to a rapid dilution.
3.3 General Assessment
3.3.1 Wadden Sea Plan Targets
The Wadden Sea Plan states that “the increasing energy production in the Wadden Sea Region, both onshore and offshore, will have several side-effects, such as increasing ship traffic in the coastal sea and cable crossing through the conservation area. Air pollution may increase as well as interference with bird flyways. The increasing ship traffic in general results in increased dredging and harbour extension” (CWSS, 2010). But no specific targets for energy production were formulated by the Wadden Sea Plan 2010 (CWSS, 2010), but as far as relevant energy production is covered within the paragraph of trilateral policy and management in the Target chapters.
First, Table 8 summarizes the Wadden Sea Plan Targets which might be affected by activities related to energy, giving examples for impact and measures taken. Then Table 9 compiles the trilateral policy and management bullets regarding aspects of energy production.
Table 8. Targets of the Wadden Sea Plan 2010 (CWSS, 2010) which might be affected by activities related to energy. Examples of impacts and mitigation measures are given.
Table 9. The trilateral policy and management bullets regarding aspects of energy production in the Wadden Sea Plan 2010 (CWSS, 2010).
3.3.2 OUV criterions of the Wadden Sea World Heritage Site
The Wadden Sea World Heritage meets three criteria of the outstanding universal value (OUV), which are outstanding geological processes, ongoing ecological and biological processes as well as vital habitats for in-situ biodiversity conservation. In Table10 these criteria and their key values are listed and examples for energy production related impacts and measure are given.
Table 10. Key values derived from the outstanding universal value criterions of the Wadden Sea World Heritage Site and how they can be affected by activities related to energy production in or adjacent to the Wadden Sea. Examples of impacts and mitigation measures are given.
3.4 Assessment of conflicts between impact factors and OUV criterions
This chapter analyses the conflicts between impact factors arising from energy production related activities in or adjacent to the Wadden Sea and the key values derived from the Outstanding Universal Value (OUV) of the World Heritage Site.
A rapid assessment matrix of conflicts is given in Table11. This matrix includes the identified energy related activities and their (potential) impacts, a description of these impacts by positive and/or negative, current and/or potential, origin (originating inside and/or outside of the property), the temporal scale (one off or rare, intermittent or sporadic, frequent or on-going), the spatial scale (restricted (i.e. affecting less than 10% of the property’s area at any one time); localised (i.e. affecting between 11 and 50%); extensive (i.e. affecting between 51-90%) or widespread (i.e. affecting between 91-100%)), and the trend (stable, increasing, decreasing). Based on an expert assessment, the matrix also shows if the effect of the identified (potential) impacts are/would be insignificant, minor, significant, or major. This assessment is based on the assumption that activities are conducted with the commonly used mitigation measures described in chapter 3.2. It is intended to represent the overall situation for the World Heritage Site.
Table 11. Matrix of conflicts between impacts related to energy production and the key values derived from the Outstanding Universal Value of the Wadden Sea World Heritage Site.
The following table shows the symbols used in the rapid assessment matrix (taken from Periodic Reporting, the trilateral science-policy matrix).
4. Recommendations
The Guiding Principle of the Trilateral Cooperation on the Protection of the Wadden Sea (TWSC) is “to achieve, as far as possible, a natural and sustainable ecosystem in which natural processes proceed in an undisturbed way”. It will not be possible to completely prevent the effects of energy production in the Wadden Sea Region. Social, economic, and ecological interests must be reconciled. There are already quite several international, trilateral, and national policies aiming at reducing impacts of energy production in general and finally, the Wadden Sea plan designates policies how to manage energy production aspects to protect the Wadden Sea.
However, the following recommendations for monitoring and research as well as management are given, where the reference to wind energy aspects is stronger due to the expected expansion.
4.1 Monitoring and research
-
In situ studies of temperature effects along cable routes are lacking to our knowledge. We recommend conducting a case study on possibly increased temperature along the cable routes and if so, also on the benthic communities.
-
Foundations of wind turbines may serve as steppingstones for alien species. As expansion of wind energy will even be accelerated in future, it is recommended to closer monitor and research on reef effects for alien species spread. Although the wind farms are located at a certain distance to the Wadden Sea, this aspect should be examined regarding its relevance to the coastal waters.
-
Knowledge especially about sublittoral habitats is rather limited. To be able to decide on new cable routes with the least ecological impact, it is necessary to have an accurate picture of where sensitive habitats are located. Knowledge should be expanded through a consistent monitoring.
-
In onshore wind farms situated in or near areas with sensitive bird species breeding, feeding or roosting areas, collision numbers and habitat displacement should be monitored. In offshore wind farms more research is recommended to enable a monitoring of collisions of birds breeding and staging in the Wadden Sea.
4.2 Management
The Netherlands, Germany and Denmark have different procedures for the planning and construction of wind farms.
-
Regarding the management of activities related to energy production, it would be ideal to use the best mitigation measures from each country in all three countries. This could include noise mitigation during pile driving, best practice for cable laying (burying depth, using laying techniques with low environmental impacts) and avoidance of light (illumination concepts) for vertical installations.
-
Grid expansion should be planned in such a way that the number of cables and cable routes be kept to a minimum. This should also consider alternative concepts such as hub solutions and cross-border solutions. This requires a more intense exchange, preferably at early stages within spatial planning processes. Cable routes should avoid sensitive areas like mussel beds, sea grass meadows and reefs as much as possible.
-
In wind farms (onshore and offshore), where high collision fatality rates are known or suspected, technical systems for automatic recognition of key bird species and event-related shutdown of wind turbines should be considered / installed.
-
Site selection of wind farms is an important factor determining the collision risk of birds. Siting should therefore be carried out with profound knowledge about bird movements, taking exchange relations with inland as well as offshore sites into account.
-
Regarding to the Wadden Sea Plan and the World Heritage Site, the Wadden Sea Countries should also stick to their commitment not to allow oil and gas exploration and exploitation within the boundaries of the area.
5. Summary
Fossil and renewable energy production takes places in proximity to or within the Wadden Sea. The development of offshore wind energy in the Netherlands, Germany and Denmark has been rapid in the last decades and will even speed up in this decade. Up to now, more than 2,000 wind turbines have been built offshore in the North Sea within the territorial waters and the EEZs of the three countries. Although the Wadden Sea conservation area is exempt from wind farm development the grid connections and interconnectors traverse the Wadden Sea as well, also affecting the area directly. Beside wind energy, the North Sea is also a place for the exploitation of fossil fuels. Crude oil is exploited from one platform in Schleswig-Holstein in the German Wadden Sea since 1987 and there are several gas mining projects in the Netherlands that extend under the Wadden Sea. For the transportation of fossils from those platforms and further offshore installations to the mainland and for interconnection of countries several cables and pipelines were installed crossing the Wadden Sea.
Although the expansion of regenerative energy is necessary to combat climate change all kinds of energy production can also have effects on the environment. Impacts are construction-related (e.g., underwater noise, disturbance of the seafloor), operational (e.g., electromagnetic fields, heat dissipation, spills or ground surface subsidence) or plant-related (collision risk for migrating birds and scaring of birds, sealing of surfaces). All forms of energy production cause an increase in ship traffic and also aerial traffic due to construction and maintenance of facilities with scaring effects for birds and marine mammals and an increased risk of accidents.
There are numerous international and national guidelines for the promotion, regulation, and spatial planning of renewable energy (construction and grid connections) and for the exploitation of oil and gas in force, both in the adjacent areas and within the territorial waters. This report gives an overview about the most important policies and regulations regarding the Wadden Sea. The Wadden Sea Plan (2010) presents the common policy that no wind turbines shall be erected in the Nature Conservation Area. This is incorporated in the Danish legal ordinance, the German national park laws and the Dutch nature reserve (PKB area). Both, for Wadden Sea Plan Targets, as well as for the OUV criteria and their key values examples for energy production related impacts and measure are given. Furthermore, an analysis on the (potential) conflicts of energy from oil and gas and renewable energy production and transportation activities with the Outstanding Universal Value (OUV) according to its criteria and the associated key values is presented.
About the authorsS. Christoph1, H. Büttger1, M. Bauer2, J. Baer1, G. Nehls1
1 BioConsult SH GmbH & Co. KG, Schobüller Str. 36, 25813 Husum, DE 2 Ministry for Energy Transition, Climate Protection, Environment and Nature Schleswig-Holstein, Mercatorstr. 3, 24106 Kiel, DE |
AknowledgementWe would like to thank numerous people in the National Park authorities or related authorities from the different regions, who have provided valuable information, namely Henrik Pind Jörgensen, Jan Havsager, Timo Hehnke, Janina Schrader, Margrita Sobottka, Claus Schulz, Dr. Gregor Scheiffarth, Dr. Klaus Janke, Dr. Karin van Thienen-Visser, Karst Jaarsma. Thanks go also to our colleagues at BioConsult SH who helped with their expertise on birds and mammals and to Lara Mielke. We also thank two reviewers for their valuable comments on the draft version of this report which improved the quality of the report considerably. |
More
Annex "Energy" ›
References
Adams, T. P., R. G. Miller, D. Aleynik, M. T. Burrows & M. Frederiksen (2014) Offshore marine renewable energy devices as stepping stones across biogeographical boundaries. Journal of Applied Ecology 51/2: 330–338.
Ashley, M. C., Mangi, S. C. & L. D. Rodwell (2014) The potential of offshore windfarms to act as marine protected areas – A systematic review of current evidence. Marine Policy 45: 301–309.
Aumüller, R., K. Boos, S. Freienstein, K. Hill & R. Hill (2011) Beschreibung eines Vogelschlagereignisses und seiner Ursachen an einer Forschungsplattform in der Deutschen Bucht. Vogelwarte 49: 9–16.
Avery, M., P. F. Springer & J. F. Cassel (1977) Weather influences on nocturnal bird mortality at a North Dakota tower. The Wilson Bulletin 89/2: 291–299.
Backer, S., M. Boekhoff, H. W. Linders & S. Sander (2013) Beitrag der Umweltbaubegleitung zur Netzanbindung von Offshore-Windparks im Wattenmeer. Presentation, ‘Umweltauswirkungen des Netzausbaus in der Nordsee’, Bundesnetzagentur, 2013, Bremen.
Bellebaum, J., A. Diederichs, J. Kube, A. Schulz & G. Nehls (2006) Flucht- und Meidedistanzen überwinternder Seetaucher und Meeresenten gegenüber Schiffen auf See. Ornitologischer Rundbrief für Mecklenburg-Vorpommern 45/Sonderheft 1: 86–90.
Bundesamt für Strahlenschutz (ed.) - BfS (2005) Grundsätze zu den Umweltauswirkungen im Zusammenhang mit elektromagnetischen Feldern und thermischen Auswirkungen der Kabelanbindung von Offshore-Windenergieparks an das Verbundstromnetz. 17
Brandt, M. J., A.-C. Dragon, A. Diederichs, A. Schubert, V. Kosarev, G. Nehls, V. Wahl, A. Michalik, A. Braasch, C. Hinz, C. Ketzer, D. Todeskino, M. Gauger, M. Laczny & W. Piper (2016) Effects of offshore pile driving on harbour porpoise abundance in the German Bight 2009 -2013. Husum (DEU), Prepared for Offshore Forum Windenergie: 46
Brenninkmeijer, A. & E. Klop (2015) Bird mortality in two Dutch wind farms: Effects of location, spatial design and interactions with powerlines. Springer/Berlin, 99–116.
Bruinzeel, L. W., J. Van Belle, L. Davids & F. J. T. van der Laar (2009) The impact of conventional illumination of offshore platforms in the North Sea on migratory bird populations. Feanwâlden
Bundesamt für Seeschifffahrt und Hydrographie - BSH (2018) Rahmenkonzept Abfall- und Betriebsstoffe für Offshore-Windparks und deren Netzanbindungssysteme in der deutschen AWZ – Version 2. Hamburg & Rostock
Bundesamt für Seeschifffahrt und Hydrographie – BSH (2020) Flächenentwicklungsplan 2020 für die deutsche Nord- und Ostsee, FEP https://www.bsh.de/DE/THEMEN/Offshore/Meeresfachplanung/Fortschreibung/_Anlagen/Downloads/FEP_2020_Flaechenentwicklungsplan_2020.pdf?__blob=publicationFile&v=6
Bundesamt für Seeschifffahrt und Hydrographie – BSH (2021) Maritime Spatial Planning. https://www.bsh.de/EN/TOPICS/Offshore/Maritime_spatial_planning/maritime_spatial_planning_node.html
Bundesministeriums der Justiz – BMJ (1992) Verordnung über das Befahren der Bundeswasserstraßen in Nationalparken im Bereich der Nordsee. https://www.gesetze-im-internet.de/npnordsbefv/BJNR002420992.html
Bundesministeriums der Justiz – BMJ (2009) Gesetz über Naturschutz und Landschaftspflege (Bundesnaturschutzgesetz - BNatSchG) https://www.gesetze-im-internet.de/bnatschg_2009/__23.html
Bundesministeriums der Justiz – BMJ (2019) Bundes-Klimaschutzgesetz. gesetze-im-internet.de/ksg/KSG.pdf
Bundesministeriums der Justiz – BMJ (2020) Raumordnungsverordnung. http://www.gesetze-im-internet.de/rov/
Bundesministeriums der Justiz – BMJ (2021a) Bundesberggesetz. https://www.gesetze-im-internet.de/bbergg/index.html
Bundesministeriums der Justiz – BMJ (2021b) Verordnung über die Raumordnung in der deutschen ausschließlichen Wirtschaftszone in der Nordsee, AWZ Nordsee-ROV http://www.gesetze-im-internet.de/awzrov/BJNR388600021.html
Bundesministeriums der Justiz – BMJ (2022) Gesetz zur Entwicklung und Förderung der Windenergie auf See. http://www.gesetze-im-internet.de/windseeg/
Bundesministerium für Umwelt, Naturschutz und nukleare Sicherheit – BMU (2019) Klimaschutzprogramm 2030. https://www.bundesregierung.de/breg-de/themen/klimaschutz/klimaschutzprogramm-2030-1673578
Bundesministerium für Wirtschaft und Klimaschutz – BMWK (2022) Erneuerbare Energien. https://www.bmwk.de/Redaktion/DE/Dossier/erneuerbare-energien.html
Bundesverband Erdgas, Erdöl und Geoenergie e.V. - BVEG (2021) Die E&P-Industrie in Zahlen. Statistischer Bericht 2020. Hannover & Berlin: 28
Camphuysen, C. J. (2019) A decline in oil rates consolidated. Monitoring and assesssment of the proportion of oiled Common Guillemot in The Netherlands. Winter 2018/19. Texel
Common Wadden Sea Secretariat – CWSS (2006) Schiermonnikoog Declaration. Eleventh Trilateral Governmental Conference on the Protection of the Wadden Sea. Common Wadden Sea Secretariat, Wilhelmshaven, Germany. https://www.waddensea-worldheritage.org/resources/2005-schiermonnikoog-declaration
Common Wadden Sea Secretariat – CWSS (2010) Wadden Sea Plan. Eleventh Trilateral Governmental Conference on the Protection of the Wadden Sea. Common Wadden Sea Secretariat, Wilhelmshaven, Germany. https://www.waddensea-worldheritage.org/resources/2010-wadden-sea-plan
Common Wadden Sea Secretariat – CWSS (2014) Toender Declaration. Ministerial Council Declaration of the 12th Trilateral Governmental Conference on the Protection of the Wadden Sea. Common Wadden Sea Secretariat, Wilhelmshaven, Germany. https://www.waddensea-worldheritage.org/resources/2014-t%C3%B8nder-decl…
Common Wadden Sea Secretariat – CWSS (2018) Leeuwarden Declaration. Ministerial Council Declaration of the 13th Trilateral Governmental Conference on the Protection of the Wadden Sea. Common Wadden Sea Secretariat, Wilhelmshaven, Germany. https://www.waddensea-worldheritage.org/resources/2018-leeuwarden-declaration
De Mesel, I., F. Kerckhof, A. Norro, B. Rumes & S. Degraer (2015) Succession and seasonal dynamics of the epifauna community on offshore wind farm foundations and their role as stepping stones for non-indigenous species. Hydrobiologia 756/1: 37–50. DOI: 10.1007/s10750-014-2157-1, ISSN: 0018-8158, 1573-5117.
De Waal, J. A., J. P. A. Roest, P. A. Fokker, I. C. Kroon, J. N. Breunese, A.G. Muntendam-Bos, A. P. Oost & G. van Wirdum (2012) The effective subsidence capacity concept: How to assure that subsidence in the Wadden Sea remains within defined limits? Netherlands Journal of Geosciences - Geologie en Mijnbouw 91/3: 385–399.
De Waal, J. A. & M. W. Schouten (2020) Regulating Subsidence and its uncertainty in the Dutch Wadden Sea. Proceedings of the International Association of Hydrological Sciences 382: 63–70.
Degraer, S., D. Carey, J. Coolen, Z. Hutchison, F. Kerckhof, B. Rumes & J. Vanaverbeke (2020) Offshore wind farm artificial reefs affect ecosystem structure and functioning: A synthesis. Oceanography 33/4: 48–57.
Dehnhard, N., J. Skei, S. Christensen-Dalsgaard, R. May, D. Halley, T. H. Ringsby & S.-H. Lorentsen (2020) Boat disturbance effects on moulting common eiders Somateria mollissima. Marine Biology 167/12.
Deutsche WindGuard GmbH( 2021) Status des Offshore-Windenergieausbaus in Deutschland. Jahr (2020). Varel: 11
Dierschke, V., R. W. Furness & S. Garthe (2016) Seabirds and offshore wind farms in European waters: Avoidance and attraction. Biological Conservation 202: 59–68. DOI: 10.1016/j.biocon.2016.08.016.
EEA (2022) Share of energy consumption from renewable sources in Europe. https://www.eea.europa.eu/ims/share-of-energy-consumption-from
Energistyrelsen (2016) Guideline for underwater noise – Installation of impact-driven piles. Kopenhagen: 7
Erbe, C., S. A. Marley, R. P. Schoeman, J. N. Smith, L. E. Trigg & C. B. Embling (2019) The Effects of Ship Noise on Marine Mammals—A Review. Frontiers in Marine Science 6: 21.
Essink, K. (1999) Ecological effects of dumping of dredged sediments; options for management. Journal of Coastal Conservation 5/1: 69–80.
European Commission – EC (2021) Commission presents Renewable Energy Directive revision. https://ec.europa.eu/info/news/commission-presents-renewable-energy-directive-revision-2021-jul-14_en
European Commission – EC (2022a) Klimazielplan für 2030. https://ec.europa.eu/clima/eu-action/european-green-deal/2030-climate-target-plan_de
European Commission – EC (2022b) Summary of the Commission assessment of the draft National Energy and Climate Plan 2021-2030. https://ec.europa.eu/energy/sites/default/files/documents/necp_factsheet_dk_final.pdf
European Commission – EC (2022c) Vertretung in Deutschland. https://germany.representation.ec.europa.eu/index_de
European Parliament Think Tank (2021) Climate action in the Netherlands: Latest state of play. europarl.europa.eu/thinktank/en/document/EPRS_BRI(2021)696184
European Union – EU (1992) Habitats Directive. EUR-Lex Document 31992L0043. https://eur-lex.europa.eu/legal-content/EN/TXT/?uri=CELEX:31992L0043
European Union – EU (2000) Water Framework Directive. EUR-Lex Document 32000L0060. https://eur-lex.europa.eu/legal-content/EN/TXT/?uri=CELEX:32000L0060
European Union – EU (2008) Marine Strategy Framework Directive (MSFD) (Directive 2008/56/EC). EUR-Lex Document 32008L0056. https://eur-lex.europa.eu/legal-content/EN/TXT/?uri=CELEX:32008L0056
European Union – EU (2009) Birds Directive. EUR-Lex Document 32009L0147. https://eur-lex.europa.eu/legal-content/EN/TXT/?uri=CELEX:32009L0147
European Union – EU (2014) EIA Directive. EUR-Lex Document 32014L0052. https://eur-lex.europa.eu/legal-content/EN/TXT/?uri=CELEX:32014L0052
Evans, W., Y. Akashi, N. S. Altman & A. M. Manville II (2007) Response of night-migrating songbirds in cloud to colored and flashing light. North American Birds 60/4: 476–488.
Evans Ogden, L. J. (1996) Collision course: the hazards of lighted structures and windows to migrating birds. Ontario
Everaert, J. (2014) Collision risk and micro-avoidance rates of birds with wind turbines in Flanders. Bird Study 61/2: 220–230.
Everaert, J. & E. W. Stienen (2007) Impact of wind turbines on birds in Zeebrugge (Belgium). Significant effect on breeding tern colony due to collisions. Biodiversity and Conservation 16/12: 3345–3359.
Fliessbach, K. L., K. Borkenhagen, N. Guse, N. Markones, P. Schwemmer & S. Garthe (2019) A ship traffic disturbance vulnerability index for northwest european seabirds as a tool for marine Spatial planning. Frontiers in Marine Science 6: 192. DOI: 10.3389/fmars.2019.00192.
Fokker, Peter. A., Freek. J. van Leijen, B. Orlic, H. van der Marel & Ramon. F. Hanssen (2018) Subsidence in the Dutch Wadden Sea. Netherlands Journal of Geosciences 97/3: 129–181. DOI: 10.1017/njg.2018.9, ISSN: 0016-7746, 1573-9708.
Freie und Hansestadt Hamburg (1990) Gesetz über den Nationalpark Hamburgisches Wattenmeer http://www.landesrecht-hamburg.de/jportal/portal/page/bshaprod.psml;jsessionid=04836C50C227E3CB7DAC3C9BC4AE1E6C.jp18?showdoccase=1&st=lr&doc.id=jlr-WattMGHArahmen&doc.part=X&doc.origin=bs
Freie und Hansestadt Hamburg (2013) Einhundertdreiunddreißigste Änderung des Flächennutzungsplans für die Freie und Hansestadt Hamburg. https://www.hamburg.de/contentblob/4258584/5505ff8406761a2210fb09e422600ce6/data/133-windenergieanlagen-text-begruendung.pdf
Freie und Hansestadt Hamburg (2019) Erste Fortschreibung des Hamburger Klimaplans https://www.hamburg.de/klimaplan/13254690/hamburger-klimaplan/
Garthe, S., P. Schwemmer, V. H. Paiva, A.-M. Corman, H. O. Fock, C. C. Voigt & S. Adler (2016) Terrestrial and marine foraging strategies of an opportunistic seabird species breeding in the Wadden Sea. PLOS ONE 11/8.
Gehring, J., P. Kerlinger & A. M. Manville (2009) Communication towers, lights, and birds: successful methods of reducing the frequency of avian collisions. Ecological Applications 19/2: 505–514.
Gesellschaft für Freilandökologie und Naturschutzplanung mbH - GfN (2012) Kabelverlegung HelWin 1. Dokumentation der biologischen Baubegleitung. Verlegung des Wattkabels.
Gill, A. B. & H. Taylor (2001) The potential effects of electromagnetic fields generated by cabling between offshore wind turbines upon Elasmobranch Fishes. Liverpool
Gimpel, A., V. Stelzenmüller, H. Haslob, J. Berkenhagen, M. F. Schupp, G. Krause & B. H. Buck (2020) Offshore-Windparks: Chance für Fischerei und Naturschutz. Braunschweig
Government of the Netherlands (2013) Energy Agreement for Sustainable Growth. https://www.government.nl/documents/publications/2013/09/06/energy-agreement-for-sustainable-growth
Government of the Netherlands (2019) Climate Agreement. https://www.government.nl/documents/reports/2019/06/28/climate-agreement
Government of the Netherlands (2022) Renewable Energy. https://www.government.nl/topics/renewable-energy/offshore-wind-energy
Grünkorn, T., A. Diederichs, D. Poszig, B. Diederichs & G. Nehls (2009) Wie viele Vögel kollidieren mit Windenergieanlagen? Natur und Landschaft 2009 84/7: 309–314.
Gyimesi, A., J. W. de Jong, A. Potiek & E. L. Bravo Rebolledo (2018) Update of KEC bird collision calculations in line with the 2030 Roadmap. Culemborg: 51
HaskoningDHV Nederland (ed.). (2021) Rapportage Onderzoek Innovatie Doorkruising Waddengebied. Groningen (NLD)
Hertz Transmission GmbH, Amprion GmbH, TenneT TSO GmbH, & TransnetBW GmbH (ed.) - NEP (2019) Netzentwicklungsplan Strom 2030. Version 2019, Zweiter Entwurf.
Hill, K., M. Rebke, C. Weiner, K. Boos, S. Freienstein, R. Aumüller & R. Hill (2014) Entwicklung und Erprobung einer Beleuchtung für Offshore-Windparks und andere Bauwerke mit geringer Attraktionswirkung auf ziehende Vögel – AVILUX. Osterholz-Scharmbeck, In Zusammenarbeit mit der REETEC GmbH, Bremen: 131
Indenrigs og Boligministeriet (2014) Bekendtgørelse om planlægning for og tilladelse til opstilling af vindmøller https://www.retsinformation.dk/api/pdf/161315
International Maritime Organization – IMO (1973) MARPOL Convention. https://www.imo.org/en/KnowledgeCentre/ConferencesMeetings/Pages/Marpol.aspx
International Maritime Organization – IMO (1983) Protocol of 1978 relating to the International Convention for the prevention of pollution from ships. https://treaties.un.org/doc/Publication/UNTS/Volume%201340/volume-1340-A-22484-English.pdf
Juretzek, C., B. Schmidt & M. Boethling (2021) Turning Scientific Knowledge into Regulation: Effective Measures for Noise Mitigation of Pile Driving. Journal of Marine Science and Engineering 9/8: 819. DOI: 10.3390/jmse9080819, ISSN: 2077-1312.
Kempf, N. (2020) Umweltbegleituntersuchungen im Bereich Mittelplate. Räumliche und zeitliche Verteilung mausernder Brandgänse im Wattenmeer (2019). Hamburg, für Wintershall Dea Deutschland AG: 23
Kirchgeorg, T., I. Weinberg, M. Hörnig, R. Baier, M. J. Schmid & B. Brockmeyer (2018) Emissions from corrosion protection systems of offshore wind farms: Evaluation of the potential impact on the marine environment. Marine Pollution Bulletin 136: 257–268. DOI: 10.1016/j.marpolbul.2018.08.058, ISSN: 0025326X.
Klima-, Energi- og Forsyningsministeriet – KEFM (2018) Energy Agreement. https://en.kefm.dk/Media/C/5/Energy%20Agreement%202018%20a-webtilg%C3%A6ngelig.pdf
Klima-, Energi- og Forsyningsministeriet – KEFM (2019) Lov om ændring af lov om fremme af vedvarende energi. LOV nr 499 af 01/05/2019. https://www.retsinformation.dk/eli/lta/2019/499
Klima-, Energi- og Forsyningsministeriet – KEFM (2020a) Bekendtgørelse af lov om elforsyning. LBK nr 119 af 06/02/2020. https://www.retsinformation.dk/eli/lta/2020/119
Klima-, Energi- og Forsyningsministeriet – KEFM (2020b) Bekendtgørelse af lov om Energinet. LBK nr 118 af 06/02/2020. https://www.retsinformation.dk/eli/lta/2020/118
Klima-, Energi- og Forsyningsministeriet – KEFM (2020c) Bekendtgørelse af lov om fremme af vedvarende energi. LBK nr 125 af 07/02/2020. https://www.retsinformation.dk/eli/lta/2020/125
Klima-, Energi- og Forsyningsministeriet – KEFM (2020d) Climate Act. https://en.kefm.dk/Media/1/B/Climate%20Act_Denmark%20-%20WEBTILG%c3%86NGELIG-A.pdf
Klima-, Energi- og Forsyningsministeriet – KEFM (2020e) Danish Climate Agreement for Energy and Industry 2020. https://en.kefm.dk/Media/C/B/faktaark-klimaaftale%20(English%20august%2014).pdf
Koschinski, S. & K. Lüdemann (2013) Entwicklung schallmindernder Maßnahmen beim Bau von Offshore-Windenergieanlagen 2013. Nehmten und Hamburg, Studie im Auftrag des Bundesamtes für Naturschutz (BfN)
Lagring, R., S. Degraer, G. de Montpellier, T. Jacques, W. Van Roy & R. Schallier (2012) Twenty years of Belgian North Sea aerial surveillance: A quantitative analysis of results confirms effectiveness of international oil pollution legislation. Marine Pollution Bulletin 64/3: 644–652.
Landesregierung Schleswig-Holstein (1999) Gesetz zum Schutze des schleswig-holsteinischen Wattenmeeres http://www.gesetze-rechtsprechung.sh.juris.de/jportal/?quelle=jlink&query=NParkG+SH&psml=bsshoprod.psml&max=true&aiz=true
Landesregierung Schleswig-Holstein (2014) Gesetz über die Landesplanung http://www.gesetze-rechtsprechung.sh.juris.de/jportal/portal/t/zg3/page/bsshoprod.psml/action/portlets.jw.MainAction?p1=0&eventSubmit_doNavigate=searchInSubtreeTOC&showdoccase=1&doc.hl=0&doc.id=jlr-PlanGSH2014rahmen&doc.part=R&toc.poskey=#focuspoint
Landesregierung Schleswig-Holstein (2021a) Landesentwicklungsplan https://www.schleswig-holstein.de/DE/landesregierung/themen/planen-baue…
Landesregierung Schleswig-Holstein (2021b) Neues Energiewende- und Klimaschutzgesetz: Landesregierung setzt auf ambitioniertere Klimaschutzziele und zusätzliche Klimaschutzmaßnahmen. In: Gesetz- und Verordnungsblatt für Schleswig-Holstein, Ausgabe Nr. 16, Kiel, 16. Dezember 2021, Seiten 1311 – 1419. https://www.schleswig-holstein.de/DE/landesregierung/ministerien-behoerden/IV/Service/GVOBl/GVOBl/2021/gvobl_16_2021.pdf?__blob=publicationFile&v=1
Landesregierung Schleswig-Holstein (2022) Raumordnungspläne https://www.schleswig-holstein.de/DE/fachinhalte/L/landesplanung/raumordnungsplaene/raumordnungsplaene.html
Madsen, P. T., M. Wahlberg, J. Tougaard, K. Lucke & P. L. Tyack (2006) Wind turbine underwater noise and marine mammals: implications of current knowledge and data needs. Marine Ecology Progress Series 309: 279–295.
Mattfeld, M., F. Ehlers & M. Reichenbach (2012) Optimising the lighting equipment on the Mittelplate drilling and production island in the German Wadden sea tidelands. Oil Gas European Magazine 38/2: 90–94.
Meißner, K., H. Schabelon, J. Bellebaum & H. Sordyl (2006) Impacts of submarine cables on the marine environment - A literature review -. Rostock: 96
Meißner, K. & H. Sordyl (2006) Literature review of offshore wind farms with regard to benthic communities and habitats. in: Ecological Research on Offshore Wind Farms: International Exchange of Experiences. PART B: Literature Review of Ecological Impacts, BfN-Skripten 186, Bonn, 284.
Mendel, B., P. Schwemmer, V. Peschko, S. Müller, H. Schwemmer, M. Mercker & S. Garthe (2019) Operational offshore wind farms and associated ship traffic cause profound changes in distribution patterns of Loons (Gavia spp.). Journal of Environmental Management 231: 429–438. DOI: 10.1016/j.jenvman.2018.10.053.
Miljøministeriet – MIM (2007) The Planning Act in Denmark https://naturstyrelsen.dk/media/nst/Attachments/planlovenpengelsk2007.pdf
Ministerie van Binnenlandse Zaken en Koninkrijksrelaties – BZK (2022) Executive Summary of the NOVI. https://www.novistukken.nl/english/default.aspx
Ministerie van Economische Zaken en Klimaat – RVO (2018) Letter to Parliament- Wind Energy at Sea 2024-2030. https://english.rvo.nl/sites/default/files/2018/03/Brief%20routekaart%20windenergie%20op%20zee%202024-2030%20-%20EN.pdf
Ministerie van Economische Zaken en Klimaat (2020) Contouren van de Energiewet (algemene toelichting). https://energiesamen.nu/media/uploads/Contouren%20Energiewet%2017%20juli%202020.pdf
Ministry of Business and Growth – EM (2016) Act on maritime spatial planning https://dma.dk/Media/637776641777785918/Act%20on%20maritime%20spatial%20planning.pdf
Müller, A. & C. Zerbs (2011) Offshore-Windparks. Messvorschrift für Unterwasserschallmessungen. Aktuelle Vorgehensweise mit Anmerkungen. Anwendungshinweise. Hamburg, Im Auftrag für das Bundesamt für Seeschifffahrt und Hydrographie
NAM B.V. (2020) Bodemdaling door aardgaswinning in Groningen, Friesland en het noorden van Drenthe. Status rapport 2020 en Prognose tot het jaar 2080. 58 50
Nederlandse Olie- en Gasportaal (2018) Mining Act of the Netherlands. https://www.nlog.nl/sites/default/files/2018-11/2018-11-04%20%20Translation%20MBW%20English%20%20MINING%20ACT%20OF%20THE%20NETHERLANDS%20PDF.pdf
Netzentwicklungsplan.de (2021) Netzentwicklungsplan 2035 https://www.netzentwicklungsplan.de/de/netzentwicklungsplaene/netzentwicklungsplan-2035-2021
Niedersachsen Ministerium für Ernährung Landwirtschaft und Verbraucherschutz – ML (2017) Neubekanntmachung der LROP-Verordnung 2017 https://www.ml.niedersachsen.de/startseite/themen/raumordnung_landesplanung/landes_raumordnungsprogramm/neubekanntmachung-der-lrop-verordnung-2017-158596.html
Niedersächsisches Vorschrifteninformationssystem - NI-VORIS (2001) Gesetz über den Nationalpark „Niedersächsisches Wattenmeer“ http://www.nds-voris.de/jportal/?quelle=jlink&query=WattenmeerNatPG+ND&psml=bsvorisprod.psml&max=true&aiz=true
Niedersächsisches Vorschrifteninformationssystem - NI-VORIS (2020a) Niedersächsisches Gesetz zur Förderung des Klimaschutzes und zur Minderung der Folgen des Klimawandels (Niedersächsisches Klimagesetz - NKlimaG). https://www.nds-voris.de/jportal/?quelle=jlink&query=KlimaSchG+ND&psml=bsvorisprod.psml&max=true&aiz=true
Niedersächsisches Vorschrifteninformationssystem - NI-VORIS (2020b) Niedersächsisches Raumordnungsgesetz. http://www.nds-voris.de/jportal/?quelle=jlink&query=RaumOG+ND&psml=bsvorisprod.psml&max=true&aiz=true
Noordzeeloket (2014) Rijksstructuurvisie Windenergie op Zee. https://www.noordzeeloket.nl/beheer/@167570/rijksstructuurvisie-0/
OLAV VILNES (2020) Danish MPs agree 6.8 GW offshore wind hike by 2030. https://www.montelnews.com/news/1125201/danish-mps-agree-68-gw-offshore-wind-hike-by-2030
OSPAR Commission (2008) OSPAR Guidance on Environmental Considerations for Offshore Wind Farm Development. http://www.ospar.org/documents?d=32631
OSPAR Commission (2012) Guidelines on Best Environmental Practice (BEP) in Cable Laying and Operation. https://www.gc.noaa.gov/documents/2017/12-02e_agreement_cables_guidelines.pdf
OSPAR Commission (2021) Strategy of the OSPAR Commission for the Protection of the Marine Environment of the North-East Atlantic 2030 [NEAES]. https://www.ospar.org/convention/strategy
Poot, H., B. J. Ens, H. de Vries, M. A. H. Donners, M. R. Wernand & J. M. Marquenie (2008) Green Light for Nocturnally Migrating Birds. Ecology and Society 13/2.
Prokop, O. (2006) Untersuchung der Auswirkungen von Kabelwärme (Offshorewindkraft-anlagen) in Küstengewässern - Einfluss eines inversen Temperaturgradienten auf die biogeochemischen Prozesse in Sedimenten. Rostock University / Thesis for a diploma, 98.
Reubens, J. T., U. Braeckman, J. Vanaverbeke, C. Van Colen, S. Degraer & M. Vincx (2013) Aggregation at windmill artificial reefs: CPUE of Atlantic cod (Gadus morhua) and pouting (Trisopterus luscus) at different habitats in the Belgian part of the North Sea. Fisheries Research 139: 28–34.
Rose, A., M. J. Brandt, R. Vilela, A. Diederichs, A. Schubert, V. Kosarev, G. Nehls, M. Volkenandt, V. Wahl, A. Michalik, H. Wendeln, A. Freund, C. Ketzer, B. Limmer, M. Laczny & W. Piper (2019) Effects of noise-mitigated offshore pile driving on harbour porpoise abundance in the German Bight 2014-2016 (Gescha 2). Husum (DEU), prepared for Arbeitsgemeinschaft OffshoreWind e.V.: 193
Russell, D. J., S. M. Brasseur, D. Thompson, G. D. Hastie, V. M. Janik, G. Aarts, B. T. McClintock, J. Matthiopoulos, S. E. Moss & B. McConnell (2014) Marine mammals trace anthropogenic structures at sea. Current Biology 24/14: R638–R639.
Schoeman, P. K. (2006) Wadden Sea Islands (The Netherlands). The Hague
Schulz, M., D. M. Fleet, K. C. J. Camphuysen, M. Schulze-Dieckhoff & K. Laursen (2017) Wadden Sea Quality Status Report. Oil Pollution and Seabirds. Wilhelmshaven, Eds.: Kloepper S. et al., Common Wadden Sea Secretariat. qsr.waddensea-worldheritage.org//reports/oil-pollution-and-seabirds: 13
Schwemmer, P., B. Mendel, N. Sonntag, V. Dierschke & S. Garthe (2011) Effects of ship traffic on seabirds in offshore waters: Implications for marine conservation and spatial planning. Ecological Applications 21/5: 1851–1860.
Skov- og Naturstyrelsen (2009) Sejlads i Vadehavet. https://naturstyrelsen.dk/media/nst/67612/vadehavet_A4.pdf
Søfartsstyrelsen (2022) Danmarks havplan https://havplan.dk/en/page/info
Staatsblad (2018) Elektriciteitswet. https://wetten.overheid.nl/BWBR0009755/2021-07-01
Staatsblad (2015a) Wet natuurbescherming. https://wetten.overheid.nl/BWBR0037552/2021-07-01
Staatsblad (2015b) Wet windenergie op zee. https://wetten.overheid.nl/BWBR0036752/2020-01-01
Staatsblad (2002) Mijnbouwwet. https://wetten.overheid.nl/BWBR0014168/2022-05-01/0
Staatsblad (2019) Klimaatwet. https://wetten.overheid.nl/BWBR0042394/2020-01-01
Stenberg, C., J. G. Støttrup, M. van Deurs, C. W. Berg, G. E. Dinesen, H. Mosegaard, T. M. Grome & S. B. Leonhard (2015) Long-term effects of an offshore wind farm in the North Sea on fish communities. Marine Ecology Progress Series 528: 257–265.
Strybny, J. & D. Schulz (2001) Sichtbarkeitsanalyse für Offshore-Windparks. Hannover.
Taormina, B., J. Bald, A. Want, G. Thouzeau, M. Lejart, N. Desroy & A. Carlier (2018) A review of potential impacts of submarine power cables on the marine environment: Knowledge gaps, recommendations and future directions. Renewable and Sustainable Energy Reviews 96: 380–391.
Thiel, M., G. Nehls, S. Bräger & J. Meissner (1992) The impact of boating on the distribution of seals and moulting ducks in the Wadden Sea of Schleswig-Holstein. Publication Series NIOZ 20: 221–233.
Umweltbundesamt (2021) Erneuerbare Energien in Deutschland. Daten zur Entwicklung im Jahr 2020. Dessau-Roßlau (DEU)
Umweltbundesamt (2022) Indikator: Erneuerbare Energien. umweltbundesamt.de/daten/umweltindikatoren/indikator-erneuerbare-energien#die-wichtigsten-fakten
UNESCO (2009) Decision 33 COM 8B.4 Natural properties - New Nominations - The Wadden Sea (Germany, Netherlands). https://whc.unesco.org/en/decisions/1946/
Van De Laar, F. J. T. (2007) Green Lights to birds. Investigation into the effect of bird-friendly lightning. Assen (NDL), NAM LOCATIE L15-FA-1: 22
Van der Spek, A. J. F. (2018) The development of the tidal basins in the Dutch Wadden Sea until 2100: the impact of accelerated sea-level rise and subsidence on their sediment budget – a synthesis. Netherlands Journal of Geosciences 97/3: 71–78.
Van Doren, B. M., K. G. Horton, A. M. Dokter, H. Klinck, S. B. Elbin & A. Farnsworth (2017) High-intensity urban light installation dramatically alters nocturnal bird migration. Proceedings of the National Academy of Sciences 114/42: 11175–11180.
Vilela, R., C. Burger, A. Diederichs, G. Nehls, F. Bachl, L. Szostek, A. Freund, A. Braasch, J. Bellebaum, B. Beckers & W. Piper (2020) Divers (Gavia spp.) in the German North Sea: Changes in abundance and effects of Offshore Wind Farms. A study into diver abundance and distribution based on aerial survey data in the German North Sea. Husum (DEU), Prepared for Bundesverband der Windparkbetreiber Offshore e.V.
VROM (2007) Ontwikkeling van de wadden voor natuur en mens https://waddenzee.nl/fileadmin/content/Dossiers/Overheid/pdf/Derde_Nota_Waddenzee_deel_4.pdf
Welcker, J. & R. Vilela (2019) Weather-dependence of nocturnal bird migration and cumulative collision risk at offshore wind farms in the German North and Baltic Seas. Husum: 70
WELT (2021) Kabelanbindung für Windparks: Inseln fürchten um Trinkwasser. https://www.welt.de/regionales/niedersachsen/article230595163/Kabelanbindung-fuer-Windparks-Inseln-fuerchten-um-Trinkwasser.html
Westerberg, H., P. Rönnbäck & H. Frimanson (1996) Effects of suspended sediments on codegg and larvae and on the behaviour of adult herring and cod. ICES CM.
WindEurope (2021) Wind energy in Europe 2020 Statistics and the outlook for 2021-2025 https://windeurope.org/intelligence-platform/product/wind-energy-in-eur…
Wolters, D. (2012) Anlage 1 zu Teil 2. Effekte und Auswirkungen der Kabelverlegung. Abschnitt Seetrasse. Arbeitshilfe Eingriffsregelung. Oldenburg (DEU), im Auftrag der TenneT Offshore GmbH
This report should be cited as: Christoph, S., Büttger H., Bauer M., Baer J. & Nehls G. (2022) Energy. In: Wadden Sea Quality Status Report. Eds.: Kloepper S. et al., Common Wadden Sea Secretariat, Wilhelmshaven, Germany. Last updated 01.02.2023. Downloaded DD.MM.YYYY. qsr.waddensea-worldheritage.org/reports/energy
All 2022 reports may be cited collectively as: Kloepper S., Bostelmann A., Bregnballe T., Busch J.A., Buschbaum C., Deen K., Domnick A., Gutow L., Jensen K., Jepsen N., Luna S., Meise K., Teilmann J. & van Wezel A. (2022) Wadden Sea Quality Status Report. Common Wadden Sea Secretariat, Wilhelmshaven, Germany. Downloaded DD.MM.YYYY. qsr.waddensea-worldheritage.org