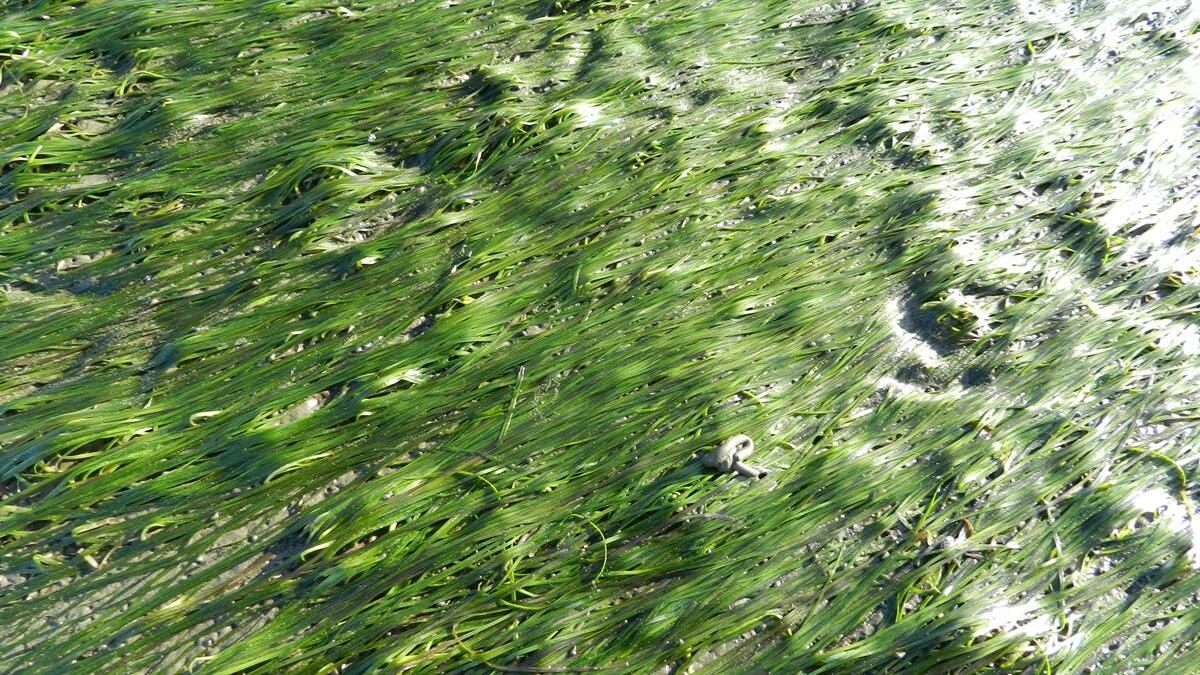
Photo: Tobias Dolch. Seagrass.
Seagrass
T. Dolch, E. O. Folmer, M. S. Frederiksen, M. Herlyn, M. M. van Katwijk, K. Kolbe, D. Krause-Jensen, P. Schmedes, E. P. Westerbeek
Published 2017
PDF1. Introduction
Seagrasses are flowering plants and often the dominant vegetation of shallow sandy bottoms in coastal areas around the world. They are one of the most productive coastal vegetation types worldwide and provide valuable ecosystem services (Orth et al., 2006). In the Wadden Sea they are a food source for brent geese and widgeon, are habitat as well as spawning and nursery ground for various animals, increase settlement of fine-grained sediments and can alter the sediment’s grain size composition (Nacken & Reise, 2000; Polte et al., 2005; van Katwijk et al., 2010).
Seagrasses are of high ecological importance for coastal ecosystems but also sensitive, especially to eutrophication, turbidity (which means light limitation), hydrodynamics (currents and waves), sediment instability, desiccation as well as to extreme changes in temperature and salinity (reviewed in e.g., Dolch et al., 2013; Folmer et al., 2016). They require sediment stability, which is also an important factor that can limit seagrass recovery (e.g., Philippart, 1994; Schanz & Asmus, 2003; Dolch & Reise 2010; Suykerbuyk et al., 2016 a, b). Seagrasses respond quickly to changing environmental conditions (Frederiksen et al., 2004a, b).
Two species of seagrass occur in the Wadden Sea: Zostera noltii and Zostera marina (Figure 1). The dwarf eelgrass Z. noltii is the most common species and is more tolerant to low tide exposure than Z. marina, which is why it grows mainly in the upper intertidal area. There are two forms of the eelgrass Z. marina: the narrow-leaved form which occurs primarily in the mid intertidal but also down to the lower intertidal. Before the wasting disease, the wide-leaved Z. marina also occurred in shallow subtidal areas (Harmsen, 1936; van Katwijk et al., 2000). Seagrass has a seasonal cycle in the Wadden Sea area: germination begins in May, maximum extent and cover of the beds are reached in August, and leaves are shed and grazed by migrant waterfowl in autumn (Vermaat & Verhagen, 1996; Nacken & Reise, 2000). The narrow-leaved Z. marina is largely annual whereas the vanished, wide-leaved, subtidal form as well as Z. noltii are largely perennial (van Katwijk et al., 1998; Zipperle et al., 2009).
Figure 1. The two intertidal seagrass species in the Wadden Sea are the narrow-leaved Zostera noltii (left) and Zostera marina (right) (Photo: T. Dolch).
In the Wadden Sea seagrass experienced two phases of dramatic decline. The ‘wasting disease’ eliminated subtidal seagrass beds of wide-leaved Zostera marina during the 1930s after which it has not recovered and is still absent from the subtidal (den Hartog, 1987). However, little is known about the historical distribution.
Intertidal beds of narrow-leaved Z. marina and Z. noltii declined during the 1970-80s presumably caused by anthropogenic eutrophication (de Jonge & de Jong, 1992). Especially the southwestern and central Wadden Sea were affected as these regions are in proximity to the big estuaries (van Katwijk et al., 2000; Dolch et al., 2013; Folmer et al., 2016). Riverine nutrient inputs to the Wadden Sea have been reduced for the last 25-30 years which has particularly benefited seagrass bed recovery in the northern Wadden Sea since the mid-1990s. The recovery was distinct here as the northern Wadden Sea is remote to the big estuaries and therefore has lower eutrophication levels than the southwestern and central Wadden Sea (van Beusekom et al., 2009 (QSR report estuaries 2009)). Seagrass in the southwestern and central Wadden Sea is at a low level and no clear signs of recovery can be observed, even though there is (modest) spontaneous, local recovery around the islands of Rottum and Griend in the Dutch Wadden Sea (Folmer et al., 2016).
2. Status and trends
With more than 200 km², the Wadden Sea comprises the largest intertidal seagrass area in Europe. However, the coverage of seagrass beds differs widely between the regions and it remains a riddle why 80 % of the seagrass occurs in the northern Wadden Sea. Therefore the status and recent development of seagrass beds are presented for specific regions individually. Additionally, Table 1 (see also Figures 9 and 10) summarize the current status of seagrass in the entire Wadden Sea region.
Table 1. Current seagrass bed area in the four regions according to the latest monitoring. It should be noted that according to the Trilateral Monitoring and Assessment Programme (TMAP) a seagrass bed is defined by a cover density of ≥ 5 %. Coverage densities below 5 % (category 1) are not monitored in Denmark and Schleswig-Holstein which is why the total bed area is composed of category 2 and 3 (coverage density ≥ 5 %). Please also note that the dataset on seagrass in the Dutch Wadden Sea is not complete.
2.1 Dutch Wadden Sea
As far as known, intertidal seagrass beds in the Dutch Wadden Sea were never as wide-spread as in the northern parts of the Wadden Sea. However, an inventory in 1972 as well as a mapping of Balgzand in 1931 and 1972 show that intertidal seagrass was far more abundant than at present day (Harmsen 1936, den Hartog & Polderman 1975; van Katwijk et al., 2006).
With 292.4 ha in 2014, the total area of seagrass beds was higher than in 2000 (224.7 ha; Figure 2). The area increased between 2000 and 2011 after which it declined marginally. However, it has to be noted that 281 ha out of 292 ha in the Dutch Wadden Sea are scattered plants (< 5 %; Table 1) and cannot suitably be called seagrass beds. The average density of seagrass has strongly decreased over the recent years and many seagrass beds in the Dutch part of the Wadden Sea have declined since 2009. While some areas with high seagrass densities were still present until 2011; in 2014 they had all disappeared (Figure 2). In this regard it has to be noted that not the whole Dutch Wadden Sea is monitored which means that new seagrass meadows, such as those currently developing at the islands of Rottum, are not included in the presented data.
On Terschelling, the area with the highest Z. noltii density (20-100 % cover) dropped from 2.68 ha in 2009 to 0.28 ha in 2014; a decline of almost 90 % (Figure 3). In areas with lower densities similar declines were observed, although not as severe. Only the area with the lowest density (0-5 % cover) has increased on Terschelling from 5.2 ha in 2009 to 6.56 ha in 2014. Along the Groningen coast, the area with the highest density of Z. noltii (20 - 100 % cover) declined even more than on Terschelling; from 7.2 ha in 2009 to 0.2 ha in 2014; a decline of 97 % (Figure 3). In the Eems-Dollard estuary however, this alarming trend was not observed and in fact, seems to have reversed. Z. noltii had not been present in the Eems-Dollard estuary since 2000, but with 0.2 ha in 2010 it has naturally resurfaced in small numbers. Moreover, in 2014 the area where Z. noltii is present has slightly increased to 0.12 ha.
Z. marina has not been reported on Terschelling since 2001 and it seems to have disappeared completely in this area. Along the Groningen coast Z. marina has been reported in very low numbers in recent years, with an exception in 2009, when it was not observed at all. Due to reintroduction activities, the species increased with 1.36 ha of the lowest density (0–5 % cover) reported in 2014. In the Eems-Dollard estuary the trend for Z. marina communities is unclear. After 2009 areas with densities higher than 5 % cover were no longer observed and seem to have disappeared completely since then. From 2010 onward a different method to assess seagrass densities was applied here. This makes comparisons of densities before and after the switch impossible without an additional retrospective analysis. In 2010, 27.24 ha of the lowest density category (0 - 5 % cover) were still reported, which declined to 10.76 ha in 2011. In 2014 this area had slightly increased to 11.84 ha.
These results confirm that the overall downward trend of the preceding years has continued in the most recent years. Only the areas with the lowest seagrass density (0-5 % cover) have increased in some places. This can be explained by the relegation of the higher density areas of the previous years to the lowest density category (0-5 % cover), since the lowest category also contains observations of a single plant per grid cell of 20 × 20 meters.
Figure 2. The development of seagrass in the Dutch Wadden Sea from 2000-2014 presented in different density classes.
Figure 3. The development of seagrass in the Dutch Wadden Sea divided into the two species Zostera noltii (Zosnol) and Zostera marina (Zosmar) and the three areas Groningen coast, Hond Paap and Terschelling.
2.2 Lower Saxony Wadden Sea, including Hamburg Wadden Sea
The latest area-wide inventory of the seagrass stock in the intertidal between Ems and Elbe estuary (Lower Saxony and Hamburg) was conducted in 2013 (Küfog et al., 2014). At this time the seagrass bed area (≥ 5 % cover) amounted to 3,760 ha which is equivalent to a doubling compared to the previous inventory from 2008 (1,879 ha; Adolph, 2010; Figure 4). This finding surpasses the formerly estimated “potential area” of 3,500 ha (Kastler & Michaelis 1997, CWSS 2006). Yet, the currently valid WFD-reference value for Lower Saxony and Hamburg – based on historical data out of the 1950s to 1960s and accomplished by recent data for the inner Ems Estuary (Hund-Paap, 2003) and Jade Bay (2008) –is 4,560 ha; it has not been met by now. The increases in seagrass area in 2013 were recorded in the entire region except the inner Ems Estuary and the tidal flats around Neuwerk and Scharhörn east of the Elbe estuary where only scarce occurrences with coverage < 5 % were found.
Both, Z. marina and Z. noltii, are present in the Lower Saxony / Hamburg part of the Wadden Sea. Z. noltii prevails and monospecific or mixed beds dominated by Z. noltii account for about 75 % (2,812 ha) of the total seagrass area. More than 50 % of the Z. noltii beds occur within or adjacent to the Jade Bay. Other prominent stands are situated in the vicinity of Borkum (Randzel) and along the mainland coastline e.g., to the south of Norderney.
Z. marina mostly occurs as isolated plant, preferably in tide pools. Only six monospecific Z. marina beds (≥ 5 % cover) were mapped. In combination with mixed beds dominated by Z. marina their area amounted to 944 ha. Z. marina is spread all over the region, but both, the largest monospecific bed (Knechtsand) and the largest mixed bed (Eversand), are situated in the outer Weser estuary.
The increase of the total seagrass bed area in 2013 was foiled by a significant all-over decrease of seagrass coverage density. While in 2008 714 ha of the seagrass bed area had a dense cover of > 20 %, it was reduced to 610 ha in 2013. As often the case for initial growth, the majority of the newly gained seagrass area was sparsely covered (< 10 %). But also long established beds suffered considerable losses of their cover density compared to former years. Since this degradation was taking place within a cover-category (5-20 %) it is not apparent in the bar-chart (Figure 4). The remarkable decline of cover has continued in the following years until now (2015) as it has been detected by annual ground surveys of six representative bed locations along the mainland coast. At sites where cover density fell below the threshold of 5 %, the area was no longer recorded as ‘seagrass bed area’. Thus the recent decline finally ended up in a decrease of seagrass bed area in 2014 and 2015 (Figure 5, see e.g., Seefelder Watt and Knechtsand).
Before the period 1993-2008, the seagrass stock along the Lower Saxony coast had gradually recovered from severe losses that occurred from the 1970s onwards (Adolph, 2010). Whether the recent decline indicates a reversal of that trend or is reflecting the natural population dynamics will turn out in the coming years.
Figure 4. Long-term development of the seagrass bed area in the Lower Saxony/Hamburg Wadden Sea from 1994 to 2013.
Figure 5. Seagrass bed area (cover ≥ 5%) at six permanent sites in the Lower Saxony Wadden Sea, mid-July to mid-September 1993/95 – 2015 in km².
2.3 Schleswig-Holstein Wadden Sea
The spatial distribution of the seagrass stock in the Schleswig-Holstein Wadden Sea is very uneven. More than 99 % of the entire seagrass stock can be found in the Northfrisian Wadden Sea, which represents the northern 2/3 of the Schleswig-Holstein Wadden Sea. The other major water body is the Dithmarscher Wadden Sea, which covers the southern 1/3. Less than 1 % of the seagrass beds are located here. Merely 0.1 % to 0.4 % of the tidal flat area in the Dithmarscher Wadden Sea was covered with seagrass in the period 2009-2015 while it was 14.0 % to 17.3 % in the Northfrisian Wadden Sea. The unequal distribution of seagrass can be ascribed to two factors: seagrass prefers sheltered habitats and occurs preferentially along the lee side of islands and high sands which are hardly existent in the Dithmarscher Wadden Sea. The influence of nutrients due to the proximity of the Dithmarscher Wadden Sea to the big estuaries most likely also hampers the development of seagrass.
Regarding the time period since the last QSR in 2009, the intertidal seagrass beds in the Schleswig-Holstein Wadden Sea show an increase in area from 13,163 ha in 2009 to 17,079 ha in 2011 (cover > 20 %). This is part of a long-term and almost steady increase that is observed since 1994 (Figure 6). From 2012 to 2015 the seagrass bed area is stable on a high level and varies between 17,842 ha and 14,274 ha with an average of 15,503 ha. This is an even higher seagrass bed area than in the late 1930s (Dolch et al., 2013).
However, it should be noted that this monitoring data mentioned above was recorded by aerial surveys by which only seagrass with a coverage of about > 20 % can be detected. Field surveys which are taking place simultaneously reveal that the total seagrass bed area (cover > 5 %) is about 10 % larger (18654 ha). Next to the large bed area, the share of very dense seagrass is also high and relatively stable: since 2009 seagrass with a cover of > 60 % makes in average almost 70 % of the bed area, ranging from 62 to 77 % (Figure 6).
The long-term development shown in Figure 6 reflects almost completely the development in the Northfrisian Wadden Sea. Since 2012 the seagrass bed area remained on a high level and seems to have reached its ecological potential in the Northfrisian Wadden Sea. Nevertheless the development is not uniform here as beds are declining in the List tidal basin while they are gaining in size at the mainland coast. In the Dithmarscher Wadden Sea the seagrass bed area fluctuated at a very low level.
Ground surveys revealed that the seagrass beds in the Schleswig-Holstein Wadden Sea are dominated by Z. noltii. In the Dithmarscher Wadden Sea all beds are composed of Z. noltii. In the Northfrisian Wadden Sea 65 % of the bed area is dominated by Z. noltii, while it is 11 % by Z. marina and 24 % are composed of both species. However, the field surveys since 2013 show a tendency that the share of Z. marina has increased. In the last three years, the bed area dominated by Z. marina has gained by 13 percentage points and the area composed of both species by 10 percentage points.
Figure 6. Long-term development of the seagrass bed area (cover ≥ 20%) in the Schleswig-Holstein Wadden Sea from 1994 to 2015.
2.4 Danish Wadden Sea
In the Danish Wadden Sea seagrasses grow in the sheltered locations leeward of the Wadden Sea islands and sandbars and partly along the mainland coast (Figure 7, left). The seagrasses present are Zostera noltii and Zostera marina with Z. noltii being the most abundant species. The Danish Wadden Sea is subdivided into four subareas, Grå Dyb, Knude Dyb, Juvre Dyb and Lister Dyb (Figure 7, right), that all host intertidal seagrasses.
During 2008-2015, the total area of Zostera in the Danish Wadden Sea ranged from a maximum of 1,140 ha in 2010 to a minimum of 670 ha in 2014. A tendency of increase from 2008 to 2010 was followed by a steady decrease from 2010 to 2014 and rather stable conditions in 2014 / 2015 with a bed area of 692 ha in 2015 (Figure 8A).
Considerable variation, however, exists between the four subareas. The two southernmost areas, Lister Dyb and Juvre Dyb, host the majority of the Danish Wadden Sea Zostera population. In Lister Dyb an increase from 2008 to 2010 was followed by decline of Zostera from 2010 to 2012 and it remained at this level until present (Figure 8B). The changes mainly happened in the more exposed parts. In contrast to the situation in Lister Dyb, the large Zostera population of Juvre Dyb has remained relatively stable during the past seven-year period except for a small decline in 2014. However, in 2015 the areal cover was back at the same level as in 2008 to 2013.
The Zostera populations of Knude Dyb and Grå Dyb in the north of the Danish Wadden Sea are much smaller than the southern populations. In Knude Dyb the Zostera area increased from 25 ha in 2008 to 65 ha in 2013 but then declined markedly in the following two years down to just 2 ha in 2015. The mapping method probably underestimated the seagrass bed area in 2014 and 2015 due to technical reasons but other surveys support that it has been declining in Knude Dyb in recent years (Bruntse pers. comm.). In Grå Dyb the Zostera beds were stable from 2009 to 2014 and then decreased from 75 ha to 47 ha between 2014 and 2015, which is the same level as it was in 2008. There is no obvious explanation for these patterns of change and it is probably a combination of natural fluctuation and variation in the mapping accuracy.
Figure 7. Distribution of seagrasses Zostera marina and Z. noltii in 2015 as interpreted from image analysis of aerial photos. Background: orthophoto mosaic from 2014 (left). Subdivision of the Danish Wadden Sea area into four basins between barrier islands and the main land.
Figure 8. Estimated distribution area of Zostera marina and Zostera noltii cover > 20 % in the Danish Wadden Sea quantified as a total (A) and by subarea (B).
2.5 Monitoring methods and data origin
As the spatial distribution as well as the size and density of the intertidal seagrass beds are extremely uneven, different monitoring methods are in use in the four regions.
In the Dutch Wadden Sea the long-term seagrass monitoring was done by means of the analysis of color-infrared (CIR) aerial photograph assessment up to 2009. After that a switch was made to a ‘grid method’ to increase the assessment accuracy of seagrass density assessment as well as the reliability of the monitoring data and to reduce costs. In 2007-2009 a pilot study in which both methods were carried out simultaneously indicated the ‘grid method’ as the best method to be used and at the same time enabled comparison between the datasets and the usability of the historical data within this new methodology. Since 2010, the grid method, in which the area to be assessed is divided in grid cells of 20 × 20 m, is used in the Dutch Wadden Sea. Each area is subsequently surveyed on foot and the occurrence and density of seagrass species (notably: Z. marina and Z. noltii) and Ruppia maritima, are recorded per grid cell. Reports of the mapping exercises as well as maps of the seagrass distribution are published on the website of Rijkswaterstaat (in Dutch).
In Lower Saxony/Hamburg, the entire seagrass stock is monitored if possible every six years within one summer since 1994. Additionally, six representative sites are investigated annually since 2008. During the field surveys which are carried out during the peak vegetation period from mid-July to mid-September, species composition (Z. marina and Z. noltii) and structure of the seagrass beds are determined. Due to the structure of seagrass beds three variables are recorded: total area (a, in km²); cover (c, in %) and proportion (c’, in %). “Cover” is defined as the area of the seagrass-covered patches in relation to the total bed area; “proportion” as the area covered by seagrass within the patches in relation to the total area of the seagrass patches.
From cover and proportion the total cover (ct) can be calculated: ct = c × c’ × 10-2. The total cover is equivalent to the cover estimated in the Schleswig-Holstein and Dutch Wadden Sea. Cover, proportion and total cover are categorized in six classes as follows: < 1 %, 1-4 %, 5-20 %, 21-40 %, 41-60 % and 61-100 %.
The total area of the beds is mapped by surrounding the ≥ 5 % borders (total cover) using a portable GPS device. Where existing, the ≥ 20 % borders (total cover) are determined along at least two transversal transects and possibly one longitudinal transect. Cover and proportion are estimated within a radius of approx. 15 m at 10 positions which are spread randomly over the whole bed area.
For determination of biomass, 10 sub-samples are taken from patches randomly distributed over the bed area. Sampling takes place by use of a sampling tube (each covering 181.5 cm²) targeted in areas of the beds which are covered by seagrass plants. Additionally in 2013 the leaf length has been measured.
Complementary to this, digital aerial photographs (e.g., CIR and RGB (red-green-blue) images) are used for the planning of field investigations and in order to improve orientation during fieldwork. This method is used since 2000 but aerial photographs are not available annually. However, a visual interpretation of analogue aerial photographs from 2008 in conjunction with ground truth data from the same year had shown that total seagrass cover must exceed 15 % to be detectable on the image with sufficient reliability (Kolbe, 2011). Currently seagrass meadows along the coast of Lower Saxony in majority do not fulfil this condition.
In the Schleswig Holstein Wadden Sea, seagrass is primarily recorded by two monitoring methods. Since 1994 an aerial mapping is carried out annually. During low tide three surveyors are mapping simultaneously the seagrass distribution directly while flying in an airplane at heights between 300 to 500 m. Each surveyor performs mapping by drawing observed seagrass beds on satellite paper map during the flight in which the beds are categorized in cover density less and more than 60 %. This method is assumed to detect seagrass beds with >20 % cover density. A synthesis from the three maps is transferred into GIS subsequently. In order to detect the seasonal development as well as the maximum size and density of the seagrass beds, this survey is carried out three times during the growth season, in June, July and August. The major advantages are that the survey is cost effective and it covers the entire area during one low tide. The drawbacks are due to the nature of this rapid assessment approach which means that the shape of recorded beds is generalized, smaller beds can be missed and experienced surveyors are needed to differentiate between green algae and seagrass.
Complementary to this, precise and high-resolution data is gained by field surveys which are carried out each year between mid-July to mid-September since 2007. This monitoring is conducted by two surveyors who map the seagrass bed with GPS devices on foot along their borders with > 5 % and > 20 % cover density in order to perform a TMAP conform mapping. Detailed information such as cover density, species composition, epiphyte cover and cover of macroalgae are also recorded from inside the bed via transversal transects. However, due to the vast tidal flat area it is not possible to ground survey the entire area within one year; therefore it is divided into six sub-areas. Each year an area covering 1/6 of the Schleswig-Holstein Wadden Sea is surveyed on foot and all seagrass beds within this area are recorded. As the 6 subareas were successively surveyed, all seagrass beds in the entire Schleswig-Holstein Wadden Sea are detected and recorded after a period of six years. The first cycle was from 2007-2012 and the second cycle started in 2013.
In the Danish Wadden Sea, the area distribution of Zostera marina and Zostera noltii is mapped on an annual basis from aerial photographs with a pixel resolution of 0.2-0.3 m. Photographs are taken in the growth season in August / September at low tide from approximately 600 m altitude. The photographs are georeferenced in GIS and areas with seagrass are detected by visual inspection. Polygons are drawn manually in GIS around the seagrass beds and the total seagrass area is quantified by summing up all polygon areas. To verify the presence of seagrass ground truthing is carried out on 7-10 transects in varying parts of the Danish Wadden Sea. Along each transect line the coverage of seagrasses, macroalgae, mussel and oyster species are registered.
The survey method has several limitations which reduce the accuracy of the mapping results. The image resolution prevents the detection of sparse seagrass cover below approximately 20 % and therefore underestimates the actual seagrass area. Furthermore, the mapping of seagrasses in tidal areas off the coast is less precise compared to seagrasses positioned closer to the shoreline due to the reduced georeferencing accuracy. More research is needed to quantify the effect of these methodological constraints.
3. Assessment
3.1 Overall assessment
Regarding the development and status of seagrass beds, the Wadden Sea can be divided into two major, ecologically comparable regions. The southwestern and central Wadden Sea stretches from the Dutch Wadden Sea along the coast of Lower Saxony to the southern shore of Eiderstedt peninsula (Figure 9). The northern Wadden Sea covers the area north of Eiderstedt peninsula to the Danish Wadden Sea (Figure 10).
Seagrass is at a rather low level in the southwestern and central Wadden Sea which means that the beds are far away from each other, generally small and rather sparsely populated. About 16 % of all Wadden Sea seagrass bed area occurs in this area but as the region is vast, seagrass covers just 1.2 % of the tidal flat area (Figure 9). Although the total seagrass area remained relatively stable in the Dutch Wadden Sea and even increased along the coast of Lower Saxony since 2009, a severe decline in seagrass density has occurred in both regions in the previous century. In the Schleswig-Holstein Wadden Sea south of Eiderstedt peninsula, seagrass bed area also fluctuates at a low level. So far no clear trend of a positive seagrass development can be seen in the southwestern and central Wadden Sea. The main reasons for the poor status could be (1) the lack of suitable, sheltered habitats, where hydrodynamic conditions are sufficiently calm and sediments stable, (2) the proximity to the estuaries with big rivers discharging nutrients, (3) and / or the lack of plants or a ‘critical mass’ (Reise & Kohlus, 2008; Dolch et al., 2013), (4) bioturbation by lugworms. The dynamic environment is reflected in the patch dynamics; in general, beds have a ‘core area’, often located on relatively stable sediment, and fluctuate yearly around it (e.g., van Katwijk et al., 2006; Valle et al., 2013). The low number of beds and their sparseness is a problem itself. Besides being a small initial population and a low seed bank for a potential recovery, positive feedback mechanisms lead to accelerated declines when seagrass cover is below 30 % (Suykerbuyk et al., 2016 a). The vast majority of seagrass beds in the southwestern and central Wadden Sea are far below this threshold value.
About 84 % of all seagrass occurs in the northern Wadden. Beds are much larger, significantly denser and occur in higher numbers here than in the southwestern and central Wadden Sea. Seagrass covers about 16 % of the tidal flat area here and it prospers especially north of Eiderstedt peninsula up to the island of Mandø, while the stock is rather variable north of Mandø (Figure 10). The northern Wadden Sea may have a higher habitat potential for seagrass as the alignment of islands and high sands provide suitable sheltered habitats against predominantly westerly winds and nutrient levels are lower. Sheltered habitats with stable sediments, which include a clay rich sediment base, seem to be a crucial prerequisite for the prospering of seagrass and also a key factor that determines the unequal distribution of beds in the Wadden Sea.
It has been argued that the reduction of nutrient loads since the mid-1980s has triggered the recovery which started in the mid-1990s (Reise & Kohlus, 2008). The increase of bed area continued until about five years ago, when seagrass seemed to have reached its ecological potential. Besides, over the last years, a distinct increase of Z. marina was observed in the northern Wadden Sea. Z. marina is often expanding in the mid to lower intertidal in areas adjoining tidal channel deltas. Based on this spatial distribution it is assumed that seeds were transported by currents to these areas and have germinated successfully there. However, the share of Z. marina has also increased in dense beds which are dominated by Z. noltii. It is possible that Z. noltii facilitates Z. marina by delaying water drainage with their dense canopy (Fox, 1996) and hereby increasing the suitability of tidal flats in the upper intertidal for Z. marina which would usually be too dry, but optimally sheltered from hydrodynamics. The dense Z. noltii cover could also retain Z. marina seeds in the beds. In any case, in the last three to four years there must have been favorable conditions for Z. marina.
Figure 9: The spatial distribution of the seagrass beds in the southwestern and central Wadden Sea, which stretches from The Netherlands (NL, data sampled in 2009), along the coast of Lower Saxony (NDS, 2013) to the south of Eiderstedt peninsula in Schleswig-Holstein (SH, 2015).
Figure 10. The spatial distribution of the seagrass beds in the northern Wadden Sea, which covers the tidal flat area north of Eiderstedt peninsula in Schleswig-Holstein (SH, 2015) and the Danish Wadden Sea (DK, 2015). Most seagrass beds occur between Eiderstedt peninsula and south of Mandø, in the sheltered lee sides of islands and high sands.
3.2 Threats
In the Wadden Sea, seagrass is generally growing in the shelter of islands and high sands (Figures 9 and 10) as it is sensitive to strong hydrodynamic forces (Fonseca & Bell 1998; Schanz & Asmus, 2003). Sediment instability is often associated with strong hydrodynamics and seagrass can also not withstand erosion or being buried by sedimentation (Cabaço & Santos, 2007; Daniell et al., 2008). The sheltered seagrass habitats are threatened, if sea level will accelerate and be accompanied by increasing hydrodynamics and sediment instability (Reise & Kohlus 2008). It can be expected that habitats which are just marginally sheltered and suitable, such as in the more open southwestern Wadden Sea, will be affected most. This can be even amplified by a changed storm pattern and increased storm intensity as a further effect of climate change. The rate and scale cannot be predicted in detail but as increased hydrodynamic forcing is expected, this will probably go along with a reduction of suitable seagrass habitats in the entire Wadden Sea, even though this effect is expected to happen slow and gradually. Climate change (see report climate change) can also result in desiccation of seagrass during hot summers and the negative impact of pathogens such as Phytophthora (Govers et al., 2016) can become more important when seagrass is stressed by heat waves.
Eutrophication (see report eutrophication) is another threat as the metabolism of seagrass is adapted to low nutrient conditions (Burkholder et al., 2007). Measures to reduce nutrient discharge are taken since the mid-1980s but the present state of eutrophication may still be detrimental for seagrass. This is especially true for the southwestern and central Wadden Sea as these regions are in proximity to the big river mouths that discharge nutrient loads. Furthermore, climate change can aggravate the effects of eutrophication. Opportunistic macroalgae benefit and prosper from nutrients (Valiela 2006; Smetacek & Zingone, 2013). Since algal growth is also favored by warm winters in terms of time scale (early growth) and spatial scale (widespread growth) they can produce considerable biomass. Dense mats of algae can be accumulated by hydrodynamics in sheltered locations where they can smother and supersede seagrass.
Epiphytic diatoms (e.g., Cocconeis sp.) are probably facilitated by eutrophication too. As they can form dense layers on the seagrass leaves, they compete for light with their host and hamper its photosynthesis. Grazing snails may fail to control these epiphytes when falling off the seagrass blades due to increased hydrodynamics (Schanz & Asmus, 2003) or when epiphyte growth is too strong.
Benthic diatoms can also benefit from eutrophication and form thick mats on the sediment surface at sheltered sites in sparsely vegetated seagrass beds. A hindering of gas exchange and microbial processes (decomposition of algal biomass) can both lead to anoxic conditions and high sulphide concentrations in the upper sediment layer which are detrimental for seagrass (Govers et al., 2014).
Light limitation due to increased turbidity by high densities of phytoplankton in the water column is another negative effect of eutrophication on seagrass growth. It might not be crucial for intertidal seagrass beds but it may prevent recolonization of subtidal areas. Turbidity can also be increased by maintenance works of fields of brushwood groins as well as by dredging and bottom disturbing fishery. Although seagrass is protected, suitable seagrass habitats are allowed to be fished for cockles in the Dutch Wadden Sea. This may hamper possible recolonization of these habitats (Folmer et al., 2016).
Especially in the southwestern and central Wadden Sea, decreasing seagrass cover density is becoming a problem, as positive feedbacks can lead to even accelerated declines of cover (Suykerbuyk et al., 2016 a). The lack of sufficiently sheltered habitats, the low number and the sparse population of beds represent also a small initial population and a low seed bank which makes a potential recovery difficult.
3.3 Actions and research
Folmer et al., (2016) developed a consensus habitat suitability model for intertidal seagrass on the basis of intertidal seagrass distributions in Schleswig-Holstein and high-resolution hydrodynamics and geomorphology for the entire Wadden Sea. The model-based on physical properties-suggests that across all regions there are substantial areas of intertidal flats that are suitable for seagrass. In the southwestern Wadden Sea there is far less intertidal seagrass than would be expected on the basis of the modeled suitability. This divergence indicates that unaccounted factors limit seagrass development in the southwestern Wadden Sea. These factors are probably both natural and anthropogenic and remain to be revealed properly. Natural factors – which have not been incorporated in the model – such as the impact of waves, the occurrence of macroalgae and the presence of the bioturbating lugworm Arenicola marina (Philippart, 1994; Valdemarsen et al., 2010, 2011) have to be analyzed. In addition, human activities such as cockle fisheries by means of hand dredging in areas adjacent to seagrass beds or in suitable habitats may also play a role. Particularly, establishment of seagrass meadows in areas that are regularly disturbed is unlikely to happen. Finally, the results from the habitat suitability model emphasize the importance of understanding the possible role of recruitment limitation by means of hydrodynamic transport. The model identified locations that may be suitable for seagrass growth but meadows fail to establish there, possibly due to the fact that seagrass propagules do not reach these areas.
New insights in various positive feedback mechanisms lead to the understanding that seagrass recovery as well as decline can accelerate (Maxwell et al., 2016). When cover of Z. noltii is below 30 %, it is not sufficiently dense to increase stabilization of sediments and gives also room to bioturbating lugworms (Arenicola marina), which both results in detrimental sediment mobility. Seagrass cover of more than 30 % can stabilize sediments, depending on the prevailing hydrodynamics and the sediment type, and leads to reduced lugworm densities, which benefits recovery and increasing cover density in seagrass beds (Suykerbuyk et al., 2016 a). The presence of plants or seeds is paramount for seagrass recovery anyway. The example from the Schleswig-Holstein area, where seagrass increases even at suboptimal sites, supports both insights.
From these findings, as well as global review, several revegetation measures have been carried out in the Dutch Wadden Sea, employing a large scale and introducing ample seed (e.g., van Duren & van Katwijk, 2013; van Katwijk et al., 2016, blog Zeegrasherstelwaddenzee). Until now, Z. marina and Z. noltii revegetations show survival and temporal expansions at several locations for three to maximum 13 years (van Katwijk et al., 2006; van Duren & van Katwijk 2013), but the restoration efforts still have to prove long-term success. Spreading of risks in addition to upscaling in combination with improved insight into habitat suitability (including variability) are likely important considerations as the success of large-scale restorations remain unpredictable in dynamic environments (Suykerbuyk et al., 2016b).
Global analysis suggest that subtidal plantings of seagrass generally show better survival and expansion than on the tidal flats (van Katwijk et al., 2016). Presently, the light climate in the subtidal is tested to see whether it could support seagrass. However until this is confirmed, Dutch Z. marina restoration efforts could better focus on the southwestern Netherlands, where chances are higher. A restoration program has actually started in Lake Grevelingen and the project will involve Lake Veere at a later stage.
3.4 QSR targets
In the two last Quality Status Reports (Reise et al., 2005 (QSR report 2004, page 201); van der Graaf et al., 2009 (QSR report 2009)) it was concluded that the target of an increased area of Zostera fields has not been met in all Wadden Sea regions. This applies especially to the southwestern and central Wadden Sea and little has changed since 2009. Even though the total seagrass bed area has increased along the coast of Lower Saxony, the overall decline in cover density that is observed in the entire region is cause for concern.
A main QSR target should be towards stimulating an increase of the bed area and cover density of Zostera in the southwestern and central Wadden Sea. The large and dense seagrass areas in the northern Wadden Sea should be preserved. Both can be achieved primarily by a reduction and removal of threats and kept free from human disturbance. We recommend further research on possible factors that may limit seagrass development and on the basis of the new insights the definition of goals for each subregion separately, such as: Which size of bed area or density should be achieved at which date? Which threats and impacts should be removed or reduced to which degree until which date? The set of sub-targets would be useful on this way.
4. Recommendations
4.1 Recommendations for monitoring & research
This report reveals that trilateral uniform monitoring methods to survey seagrass or at least monitoring methods that produce directly comparable data are still not implemented and that the quality and reliability of data varies. This makes data comparison between the individual Wadden Sea regions difficult. In order to increase data quality and to make survey results from different subregions directly comparable, it is strongly recommended to agree on methods that produce comparable data and intervals that are then consistently implemented. It is understood that the seagrass situation is different in each subregion and monitoring methods were developed and optimized over the years to fulfil site-specific circumstances. Nevertheless, an approach for harmonization with the objective of data with a better comparability is strongly recommended and options should be discussed. We also suggest installing a TMAP database where survey data should be uploaded regularly. This would benefit data availability, ensure a consistent monitoring and reduce time gaps between QSR reports.
Furthermore, it is advised to discuss on a trilateral base which environmental variables should be included in the monitoring in order to assess the ecological status of seagrass beds. We suggest including information on hydrodynamic impact, sediment properties (e.g., composition, stability, nutrient content), seagrass seed production or information on biota (e.g., microalgae cover, epiphytes and lugworm density).
Regarding the accuracy of hydrodynamical models for currents and waves, we suggest to employ empirical validation by means of field measurements in the intertidal and shallow subtidal zones.
In order to increase the success of seagrass recovery and natural recolonization, it is recommended to identify and name the most important local stress factors to seagrass for each area (tidal basin) in the Wadden Sea regions (Maxwell et al., 2016).
Phytophthora disease might be a potential threat to seagrass. We suggest collecting seagrass samples during fieldwork which are then checked for Phytophthora in order to get information about an area-wide distribution.
The use of innovative techniques, such as UAV (unmanned aerial vehicle) e.g., drones might be considered for monitoring of areas hard to access and for harmonization of monitoring across regions. This requires insights into the possibilities of detecting and estimating densities of seagrass with different setups. Important aspects to consider are the effectivity of (multispectral) cameras, flight altitudes and flight speed on different types of sediment and the occurrence of macroalgae. For example, to which degree is the detection and assessment of seagrass limited by the contrasts with the sediment or by the presence of microphytobenthos and macroalgae? Still there is only little literature about the impact of drones on birds and seals. According to personal observation, it varies between species and also between seasons in if and how they react, e.g., oystercatchers react in great numbers, terns usually react when not yet on eggs and seals hardly respond, even though the ones that live in more disturbed areas seem to be more sensitive (Heusinkveld pers. comm.). Furthermore, the shape of the drone is also an important aspect as drones in the shape of an airplane resemble big birds and generally cause more disturbance than more symmetrical drones. More information on this topic is necessary.
The seagrass habitat model by Folmer et al. (2016) revealed that far less intertidal seagrass occurs in the southwestern and central Wadden Sea than what would be expected on the basis of the modeled suitability. This divergence indicates that factors unaccounted for by the model limit seagrass development in the southwestern Wadden Sea. We strongly propose further development of the monitoring effort in combination with targeted research and systematic conservation planning. This should be discussed on a trilateral basis. In this regard it is e.g., also important to understand the possible role of recruitment limitation by means of hydrodynamic transport. Particularly, locations may be suitable for seagrass growth but meadows may fail to establish due to the fact that seagrass propagules do not reach the suitable areas. Insights into the habitat suitability, actual distributions and hydrodynamic connectivity may be useful to support targeted management of intertidal flats. It would be also useful to know how natural and anthropogenic conditions are at (newly) occupied locations compared to other suitable locations where seagrass meadows are not (yet) developing. In this regard the role of human activities (such as cockle fisheries) in areas adjacent to seagrass beds or in suitable habitats has also to be investigated.
4.2 Recommendations for management
Based on the results from the various Wadden Sea regions and recent reviews on seagrass ecosystem management recommendations (e.g., van Katwijk et al., 2016; Maxwell et al., 2016; Suykerbuyk et al., 2016a, b), the following recommendations for improving the Wadden Sea seagrass communities are highlighted:
- Consider reduction (if possible removal) of threats as the first step and the top priority. Therefore, it is important to identify local stressors in the forefront (see above). The development in the northern Wadden Sea suggests that some habitats and populations still have the natural resilience to restore or approach their former states of before the anthropogenic pressures;
- It has to be assumed that nutrient discharges to the Wadden Sea and hence eutrophication levels are still too high (report eutrophication) and therefore limit the growth and distribution of seagrass. It is recommended to continue the reduction of nutrient loads to lower stress for seagrass especially in the southwestern and central Wadden Sea. The example from the northern Wadden Sea shows that natural recovery can successfully happen once a (major) threat is removed or reduced even though a temporal delay (in this case about 10 years) has to be expected;
- Protection of areas larger than the present seagrass beds; both a larger buffer zone around the beds to allow for expansion, as well as ‘high potential’ areas on the basis of habitat suitability maps;
- Furthermore we recommend a critical review of dredging and dumping activities of sediments. They should take place on sites remote from beds so that seagrass neither gets buried with sediments nor gets affected by the plume that increases turbidity. Bottom disturbing fishing should also be managed to avoid turbidity and extra sediment instability. This includes cockle fisheries by means of hand dredging, which is an activity that would remove standing seagrass plants and possibly prevents establishment. Although cockle dredging is illegal inside existing seagrass beds, it is currently allowed inside a major part of the suitable habitat in the Dutch Wadden Sea. As mechanical damage of seagrass sites can also be caused by coastal protection measures, such as maintenance works of fields of brushwood groins, collaboration between coastal and nature protection should also be improved;
- Restoration activities might be considered as a kick-off, as e.g., seagrass cannot recolonize tidal flats when the stock has gone completely and no initial population is available. However, restoration efforts should only be initiated when there is clear understanding of what limits seagrass growth. Threats, the reasons, and impacts of a measure have to be well-thought-out. Goals for restoration have to be defined beforehand, the sites carefully selected and the measures should help to restore a former state or support nature development in its initial phase.
5. Summary
Most seagrass beds in the northern Wadden are large, dense, occur in high numbers and cover on average about 16 % of the tidal flat area. After an almost steady increase in bed area since the mid-1990s, the majority of the seagrass populations seemed to have reached its optimum extent in the last five years. An increase of Z. marina has been observed for the last four years which might be due to germination facilitated by dense beds of Z. noltii.
In contrast, seagrass is at a rather low level in the southwestern and central Wadden Sea. The beds are far away from each other, generally small, rather sparsely populated and cover just 1.2 % of the tidal flat area. Although the total seagrass area remained relatively stable or even increased since 2009, a severe decline in seagrass density is observed. Seagrass in the southwestern and central Wadden Sea is still at a low level and no clear sign of recovery can be observed. However, it should be noted that new seagrass meadows have appeared south of the Rottums and near Griend in the Dutch Wadden Sea.
In the southwestern and central Wadden Sea, seagrass beds are in proximity to the big river mouths, which may be a crucial reason for their poor status next to the lack of sufficiently sheltered habitats. The riverine nutrient discharges are regarded to be still too high and climate change may aggravate effects of eutrophication by e.g., promoting the growth of opportunistic macroalgae or epiphytes. Sea level rise and a changed storm pattern are also a result of climate change and lead to seagrass threats: strong hydrodynamics and associated sediment instability. Both are expected to increase and thereby likely to reduce suitable seagrass habitats in the Wadden Sea. Besides these external impacts, the decreasing seagrass cover density of already sparsely populated beds as well as the low number of seagrass beds itself, is a problem as it hinders recovery or can even lead to accelerated decline. When cover density of Zostera noltii is below 30 %, feedback mechanisms can lead to accelerated decline while cover density of more than 30 % can result in accelerated recovery. Next to the cover density, presence of seeds is also paramount for seagrass recovery as well as the reduction of threats (see reports on eutrophication, climate change, climate ecosystem, geomorphology).
The reduction or removal of threats should be given top priority in order to improve seagrass status. Therefore it is necessary to identify the most important local stress factors in each region. Natural recovery without human participation is recommended but in areas where the natural capacity to restore or approach a former state is lost, restoration activities might be considered. Goals for restoration have to be defined beforehand, the reasons why a measure should be carried out and the impacts of a measure have to be well-thought-out, and the sites carefully selected.
In order to increase the quality of information on the seagrass status and to make survey results from each Wadden Sea region directly comparable, it is strongly recommended to agree on a harmonization of monitoring methods and intervals that are then consistently implemented. The use of innovative techniques such as drones might be also considered for monitoring. Furthermore, we suggest installing a TMAP database where survey data should be uploaded regularly.
A consensus habitat suitability model for intertidal seagrass suggests that there are substantial areas of intertidal flats suitable for seagrass which are not occupied yet. This likely indicates that factors unaccounted by the model limit seagrass development and in order to identify them, it is recommended to revise monitoring and include new parameters. Further insights into habitat suitability, actual distributions and hydrodynamic connectivity may be useful to support targeted management of intertidal flats.
About the authors T. Dolch1, E. O. Folmer2, M. S. Frederiksen3, M. Herlyn4, M. M. van Katwijk5, K. Kolbe4, D. Krause-Jensen6, P. Schmedes3, E. P. Westerbeek7 1 Alfred-Wegener-Institut Helmholtz-Zentrum für Polar und Meeresforschung (AWI), Wadden Sea Station Sylt, Hafenstraße 43, 25992 List/Sylt, DE 2 Royal Netherlands Institute for Sea Research (NIOZ), P.O. Box 59, 1790 AB Den Burg, Texel, The Netherlands, NL 3 Environmental Protection Agency, Haraldsgade 53, DK-2100 København, DK 4 NLWKN-Lower Saxony Water Management, Coastal Defence and Nature Conservation Agency, Am Sportplatz 23, 26506 Norden, DE 5 Department of Environmental Science, Radboud University Nijmegen, P.O. Box 9010, NL-6500 GL Nijmegen, NL 6 Department of Bioscience-Marine Ecology, Aarhus University, Vejlsøvej 25, 8600 Silkeborg, DK 7 formerly Rijkswaterstaat Zee & Delta, NL |
AcknowledgementsWe thank Karsten Reise, Christian Buschbaum, Hanne Fogh Vinther and Grethe Bruntse for collecting and providing seagrass data. Dick de Jong, Christian Buschbaum and Karsten Reise are acknowledged for their very helpful comments on this report and Jannes Heusinkveld for his information on drones. |
References
Adolph, W. (2010) Praxistest Monitoring Küste (2008. Seegraskartierung - Gesamtbestandserfassung der eulitoralen Seegrasbestände im Niedersächsischen Wattenmeer und Bewertung nach EG-Wasserrahmenrichtlinie. NLWKN Küstengewässer und Ästuare 2/2010, 52 pp.
Burkholder, J.M., D.A. Tomasko & B.W. Touchette (2007) Seagrasses and eutrophication. Journal of Experimental Marine Biology and Ecology 350: 46-72
Cabaço, S. & R. Santos (2007) Effects of burial and erosion on the seagrass Zostera noltii. Journal of Experimental Marine Biology and Ecology 340: 204-212
CWSS (Common Wadden Sea Secretariat) (2006) Monitoring of Seagrass in the Wadden Sea. HARBASINS report of the TMAP ad hoc working group Seagrass. 28.8.2006, 19 pp.
Daniell, J.J., P.T. Harris, M.G. Hughes, M. Hemer & A. Heap (2008) The potential impact of bedform migration on seagrass communities in Torres Strait, northern Australia. Continental Shelf Research 28 (16): 2188-2202
den Hartog, C. & P.J.G. Polderman (1975) Changes in the seagrass populations of the Dutch Waddenzee. Aquatic Botany 1: 141-147
den Hartog, C. (1987) Wasting disease and other dynamic phenomena in Zostera beds. Aquatic Botany 27: 3-14
de Jonge, V.N. & D.J. de Jong (1992) Role of tide, light and fisheries in the decline of Zostera marina L. in the Dutch Wadden Sea. Netherlands Institute for Sea Research Publication Series 20: 161-176
Dolch, T. & K. Reise (2010) Long-term displacement of intertidal seagrass and mussel beds by expanding large sandy bedforms in the northern Wadden Sea. Journal of Sea Research 63: 93-101
Dolch T., C. Buschbaum & K. Reise (2013) Persisting intertidal seagrass beds in the northern Wadden Sea since the 1930s. Journal of Sea Research 82: 134-141
Frederiksen, M., D. Krause-Jensen, M. Holmer & J.S. Laursen (2004 a) Long-term changes in area distribution of eelgrass (Zostera marina) in Danish coastal waters. Aquatic Botany 78: 167-181
Frederiksen, M., D. Krause-Jensen, M. Holmer & J.S. Laursen (2004 b) Spatial and temporal changes in eelgrass (Zostera marina) landscapes: influences of physical settings. Aquatic Botany 78: 147-165
Folmer, E.O., J.E.E. van Beusekom, T. Dolch, U. Gräwe, M.M. van Katwijk, K. Kolbe & C.J.M. Philippart (2016) Consensus forecasting of intertidal seagrass habitat in the Wadden Sea. Journal of Applied Ecology, doi: 10.1111/1365-2664.12681
Fonseca, M.S. & S.S. Bell (1998) Influence of physical settings on seagrass landscapes near Beaufort, North Carolina, USA. Marine Ecology Progress Series 171: 109-121
Fox, A.D. (1996) Zostera exploitation by Brent Geese and Wigeon on the Exe Estuary, southern England. Bird Study 43: 257–268
Govers. L.L., J.H.F. de Brouwer, W. Suykerbuyk, T.J. Bouma, L.P.M. Lamers, A.J.P. Smolders & M.M. van Katwijk (2014) Toxic effects of increased sediment nutrient and organic matter loading on the seagrass Zostera noltii. Aquatic Toxicology 155: 253-260
Govers, L.L., W. Man in 't Veld, J.P. Meffert, T.J. Bouma, P.C.J. van Rijswick, J.H.T. Heusinkveld, R.J. Orth, M.M. van Katwijk & T. van der Heide (2016) Marine Phytophthora species can hamper conservation and restoration of vegetated coastal ecosystems (Proceedings of the Royal Society B 283: 20160812). http://dx.doi.org/10.1098/rspb.2016.0812
Harmsen, G.W. (1936) Systematische Beobachtungen der Nordwest-Europäischen Seegrasformen. Nederlands Kruidkundig Archief, 46: 852–877
Kastler, T. & H. Michaelis (1997) Der Rückgang der Seegrasbestände im niedersächsischen Wattenmeer. Bericht Forschungsstelle Küste 41, Norderney: 119-139
Kolbe, K. (2011) Erfassung der Seegrasbestände im niedersächsischen Wattenmeer über visuelle Luftbildinterpretation - 2008. NLWKN, Küstengewässer und Ästuare 4. 35 pp.
KÜFOG GmbH, J. Steuwer & S. Tyedmers (2014. Eulitorale Seegrasbestände im niedersächsischen Wattenmeer 2013. Gesamtbestandserfassung und Bewertung nach EG Wasserrahmenrichtlinie. - NLWKN Küstengewässer und Ästuare Band 8, 64 pp.
Maxwell, P.S., J.S. Eklöf, M.M. van Katwijk, K.R. O’Brien, M. de la Torre-Castro, C. Boström, T.J. Bouma, D. Krause-Jensen, R.K.F. Unsworth, B.I. van Tussenbroek & T. van der Heide (2016) The fundamental role of ecological feedback mechanisms for the adaptive management of seagrass ecosystems – a review. Biological Reviews, doi: 10.1111/brv.12294
Nacken, M. & K. Reise (2000) Effects of herbivore birds on intertidal seagrass beds in the northern Wadden Sea. Helgoland Marine Research 54: 87-94
Orth, R.J., T.J.B. Carruthers, W.C. Dennison, C.M. Duarte, J.W. Fourqurean, K.L. Heck, A.R. Hughes, G.A. Kendrick, W.J. Kenworthy, S. Olyarnik, F.T. Short, M. Waycott & S.L. Williams (2006) A global crisis for seagrass ecosystems. Bioscience 56: 987-996
Philippart, C.J.M. (1994) Interactions between Arenicola marina and Zostera noltii on a tidal flat in the Wadden Sea. Marine Ecology Progress Series 111: 251-257
Polte, P., A. Schanz & H. Asmus (2005) The contribution of seagrass beds (Zostera noltii) to the function of tidal flats as a juvenile habitat for dominant, mobile epibenthos in the Wadden Sea. Marine Biology 147: 813-822
Reise, K., Z. Jager, D. de Jong, M.M. van Katwijk & A. Schanz (2005) Seagrass. Wadden Sea Quality Status Report 2004. In: Essink, K., C. Dettmann, H. Farke, K. Laursen, G. Lüerßen, H. Marencic & W. Wiersinga (Eds.), Common Wadden Sea Secretariat, Wilhelmshaven, Germany: 155-160
Reise, K. & J. Kohlus (2008) Seagrass recovery in the Northern Wadden Sea? Helgoland Marine Research 62: 77-84
Schanz, A. & H. Asmus (2003) Impact of hydrodynamics on development and the morphology of intertidal seagrasses in the Wadden Sea. Marine Ecology Progress Series 261: 123-134
Smetacek, V. & A. Zingone (2013) Green and golden seaweed tides on the rise. Nature 504: 84-88
Suykerbuyk, W., T.J. Bouma, L.L. Govers, K. Giesen, D.J. de Jong, P.M.J. Herman, A.J. Hendriks & M.M. van Katwijk (2016 a) Surviving in changing seascapes: Sediment dynamics as bottleneck for long-term seagrass presence. Ecosystems 19: 296-310
Suykerbuyk, W., L.L. Govers, T.J. Bouma, W.B.J.T. Giesen, D.J. de Jong, R. van de Voort, K. Giesen, P.T. Giesen & M.M. van Katwijk (2016 b) Unpredictability in seagrass restoration: analysing the role of positive feedback and environmental stress on Zostera noltii transplants. Journal of Applied Ecology 53: 774-784
Valdemarsen, T., P. Canal-Vergés, E. Kristensen, M. Holmer, M.D. Kristiansen & M.R. Flindt (2010) Vulnerability of Zostera marina seedlings to physical stress. Marine Ecology Progress Series 418: 119–130
Valdemarsen, T., K. Wendelboe, J.T. Egelund, E. Kristensen & M.R. Flindt (2011) Burial of seeds and seedlings by the lugworm Arenicola marina hampers eelgrass (Zostera marina) recovery. Journal of Experimental Marine Biology and Ecology 410: 45–52
Valiela, I. (2006) Global coastal change. Blackwell Publ., Malden USA. 368 pp.
Valle M., M.M. van Katwijk, D.J. de Jong, T.J. Bouma, A.M. Schipper, G. Chust, B.M. Benito, J.M. Garmendia & A. Borja (2013) Comparing the performance of species distribution models of Zostera marina: implications for conservation. Journal of Sea Research 83: 56-64
van Beusekom, J.E.E., P.V.M. Bot, J. Carstensen, J.H.M. Goebel, H. Lenhart, J. Pätsch, T. Petenati, T. Raabe, K. Reise & B. Wetsteijn (2009) Eutrophication. Quality Status Report 2009 - Wadden Sea Ecosystem No. 25 - Thematic Report No. 12. In: Marencic, H. & J. de Vlas (Eds.). Common Wadden Sea Secretariat, Wilhelmshaven, Germany, Trilateral Monitoring and Assessment Group
van der Graaf, S., I. Jonker, M. Herlyn, J. Kohlus, H. Fogh Vinther, K. Reise, D.J. de Jong, T. Dolch, G. Bruntse & J. de Vlas (2009) Seagrass. Quality Status Report 2009 - Wadden Sea Ecosystem No. 25 - Thematic Report No. 12. In: Marencic, H. & J. de Vlas (Eds.), Common Wadden Sea Secretariat, Wilhelmshaven, Germany, Trilateral Monitoring and Assessment Group.
van Duren, L.A. & M.M. van Katwijk (2013) Eelgras restoration in the Dutch Wadden Sea, results 2013. Project 12038902, Deltares Delft, The Netherlands, p 1-19
van Katwijk, M.M., G.H.W. Schmitz, L.S.A.M. Hanssen & C. den Hartog (1998) Suitability of Zostera marina populations for transplantation to the Wadden Sea as determined by a mesocosm shading experiment. Aquatic Botany 60: 382-305
van Katwijk, M.M., D.C.R. Hermus, D.J. de Jong & V.N. de Jonge (2000) Habitat suitability of the Wadden Sea for Zostera marina restoration. Helgoland Marine Research 54: 117-128
van Katwijk, M.M., G.W. Geerling, R. Rasin, R. van 't Veer, A.R. Bos, D.C.R. Hermus, M. van Wieringen, Z. Jager, A.H. Groeneweg, P.L.A. Erftemeijer, T. van der Heide & D.J. de Jong (2006) Macrophytes in the western Wadden Sea: monitoring, invasion, transplantations, dynamics and European policy. In: K. Laursen, H. Marencic et al. (Eds.), Proceedings of the 11th International Scientific Wadden Sea Symposium. Esbjerg, Denmark
van Katwijk, M.M., W. Suykerbuyk, D.C.R. Hermus & A.R. Bos (2010) Sediment modification by Zostera marina beds: muddification and sandification induced by plant cover and environmental conditions. Estuarine Coastal and Shelf Science 89: 175-181
van Katwijk, M.M., A. Thorhaug, N. Marbà, R.J. Orth, C.M. Duarte, G.A. Kendrick, I.H.J. Althuizen, E. Balestri, G. Bernard, M.L. Cambridge, A. Cunha, C. Durance, W. Giesen, Q. Han, S. Hosokawa, W. Kiswara, T. Komatsu, C. Lardicci, K.S. Lee, A. Meinesz, M. Nakaoka, K.R. O'Brien, E.I. Paling, C. Pickerell, A.M.A. Ransijn & J.J. Verduin (2016) Global analysis of seagrass restoration: the importance of large-scale planting. Journal of Applied Ecology 53: 567-578
Vermaat, J.E. & F.C.A. Verhagen (1996) Seasonal variation in the intertidal seagrass Zostera noltii Hornem, Coupling demographic and physiological patterns. Aquatic Botany 52: 259-281
Zipperle, A.M., J.A. Coyer, K. Reise, W.T. Stam & J.L. Olsen (2009) Evidence for persistent seed banks in dwarf eelgrass Zostera noltii in the German Wadden Sea. Marine Ecology Progress Series 380: 73-80
This report should be cited as: Dolch T., Folmer E.O., Frederiksen M.S., Herlyn M., van Katwijk M.M., Kolbe K., Krause-Jensen D., Schmedes P. & Westerbeek E.P. (2017) Seagrass. In: Wadden Sea Quality Status Report. Eds.: Kloepper S. et al., Common Wadden Sea Secretariat, Wilhelmshaven, Germany. Last updated 21.12.2017. Downloaded DD.MM.YYYY. qsr.waddensea-worldheritage.org/reports/seagrass
All 2017 reports may be cited collectively as: Kloepper S., Baptist M. J., Bostelmann A., Busch J.A., Buschbaum C., Gutow L., Janssen G., Jensen K., Jørgensen H.P., de Jong F., Lüerßen G., Schwarzer K., Strempel R. & Thieltges D. (2017) Wadden Sea Quality Status Report. Common Wadden Sea Secretariat, Wilhelmshaven, Germany. Downloaded DD.MM.YYYY. qsr.waddensea-worldheritage.org