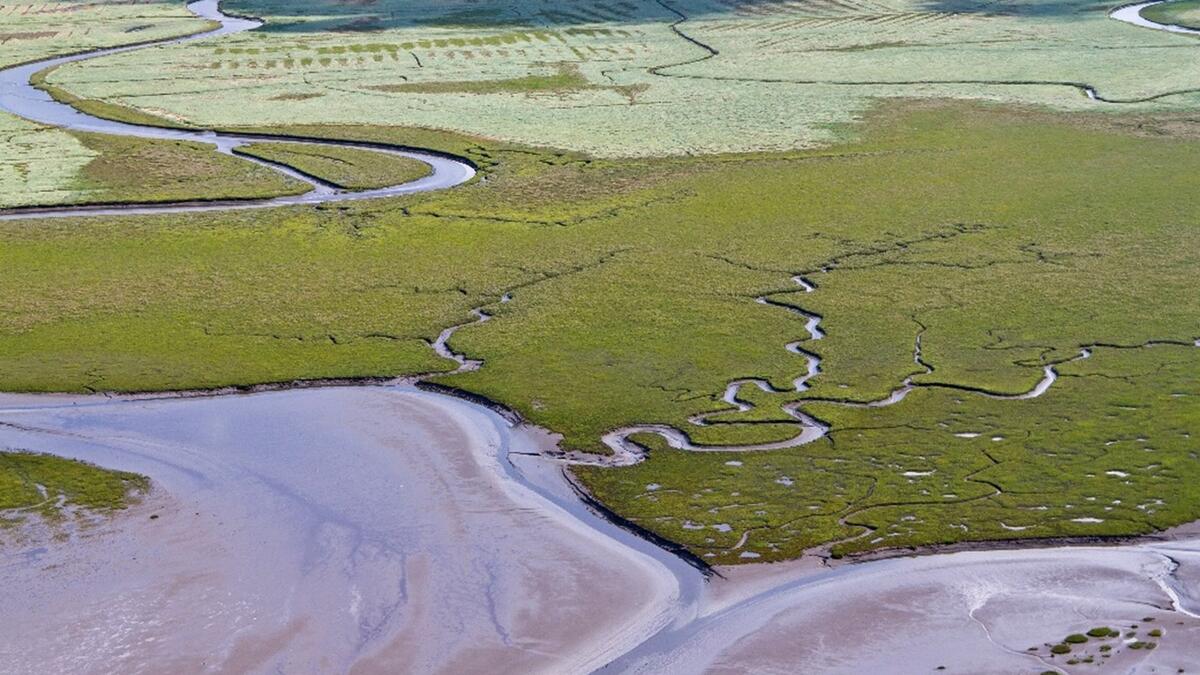
Photo: Natural marsh development in front of man-made foreland-type marshes at Dieksanderkoog, southern Schleswig-Holstein. © Martin Stock.
Salt marshes
K. Elschot, P. Esselink, J. Frikke, K. Jensen, N. Janinhoff-Verdaat, M. Paarup Thomsen, M. Padlat, J.T. van der Wal, W.E. van Duin, M.E.B. van Puijenbroek, F. Rupprecht, M. Stock
Published 2024
1. Introduction
Coastal salt marshes are areas vegetated by herbs, grasses, or low shrubs, which are subject to periodic flooding by saline water (Odum, 1988; Adam, 1990). They reach their greatest extent along low-energy coasts where wave action is limited and mud can accumulate (Allen & Pye, 1992; Balke et al., 2016). In the Wadden Sea, salt marshes form the upper part of the intertidal zone and extend vertically from well below the mean high-tide level up to the highest water mark. Various human activities have a direct or indirect impact on salt marshes, such as land claim, coastal defence, land use, pollution, and eutrophication. In addition, they can be vulnerable to an accelerated sea-level rise caused by global warming (Kirwan et al., 2010), whereas elevated atmospheric CO2-concentrations and rising temperatures also affect ecological processes within salt marshes (Drake, 2014).
According to the EU Habitat Directive, salt marshes include the habitat types 1310 (Salicornia and other annuals colonising mud and sand), 1320 (Spartina swards), and 1330 (Atlantic salt meadows), for which national conservation objectives have been elaborated.
Trilateral policy and management
Almost all salt marshes in the Wadden Sea area are part of Natura 2000 sites. In addition to the national legislation and nature protection regulations in the Netherlands, Germany, and Denmark, the trilateral 2010 Wadden Sea Plan (WSP) provides the framework for the management of the entire area (CWSS, 2010).
To assess the targets defined in the WSP (see section 3.1 in this report), the following topics will be discussed:
- Present status and trends of the Wadden Sea salt marshes;
- Artificial drainage and vegetation management;
- Marsh surface elevation change in relation to sea-level rise;
- Ecological restoration, expansion, and construction of salt marshes;
- An assessment of the status and a Wadden Sea Plan Target evaluation with recommendations.
2. Status and trends
2.1 Types and total area
Salt marshes form the upper part of the intertidal zone, the interface between land and sea, and are strongly controlled by geomorphological, physical, and biological processes, such as sedimentation in interaction with the vegetation, tidal regime, and wind-wave pattern. They constitute a habitat for a wide range of organisms.
During the most recent monitoring period between 2015 and 2021, salt marshes in the Wadden Sea were reported to cover more than 42,000 ha (Table 1). This area represents about 20% of the total area of coastal salt marshes in Europe (Doody, 2008). Although the extent of salt marshes in the Wadden Sea is considerable, it is notable that these areas were more extensive in the past than they are today (Wiersma et al., 2009; Vos & Knol, 2015). The major cause of their decline during historical times was land-acquisition for agriculture and, more recently, coastal-protection measures. In the Wadden Sea, loss of salt-marsh area through human intervention continued throughout the twentieth century until the paradigm shift from exploitation to conservation in the 1970s and following decades.
In terms of geomorphology, five types of salt marsh can be distinguished in the Wadden Sea (Figure 1): (i) back-barrier marshes, (ii) green beaches, (iii) chenier-protected marshes, (iv) foreland-type marshes, and (v) Hallig salt marshes. The here reported extent of salt marshes and their zonation are based on vegetation and habitat mapping using the TMAP typology (Petersen et al., 2014).
Back-barrier salt marshes are in principle natural marshes. They develop in the lee of a barrier or dune system (Olff et al., 1997; Bakker et al., 2023), and usually have coarse-grained soils (Oost et al., 2021). The construction of artificial dune ridges in the past has stimulated the development of back-barrier salt marshes, such as the Boschplaat salt marsh on Terschelling (Netherlands) and the salt marsh of the Skallingen peninsula (Denmark). The presence of extensive artificial dune ridges explains why the extent of back-barrier marshes in the Dutch Wadden Sea today is much larger than their historic reference values (Dijkema, 1987a). On some German islands, such as Norderney and Juist, parts of the back-barrier salt marshes have been artificially drained. A side effect of both the artificial ridges and the draining is that natural dynamics are reduced, and vegetation succession is accelerated (Esselink et al., 2009; de Groot et al., 2016).
Green beaches may develop in the seaward area of a dune ridge (Bakker et al., 2023). In terms of vegetation, green beaches cannot be considered entirely as salt marsh, because they mostly form a mosaic of salt marsh and dune vegetation (van Puijenbroek et al., 2021). Therefore, at the landscape level, they are a transition between the beach, the dune system, and salt marshes. Since green beaches are not distinguished as a landform, halophytic vegetation of green beaches is merged with back-barrier marshes into one group, viz.: barrier-connected salt marshes (see, e.g., Table 1).
Chenier-protected salt marshes are located on chenier islands (Box 1). These marshes were recently defined by Govers et al. (2021). In this report, we therefore added chenier-protected marshes as a new geomorphological distinct salt-marsh type for the Wadden Sea, which was not included in previous QSRs.
Foreland-type salt marshes on the mainland are largely man-made, because their development has been promoted by the construction of sedimentation fields and ditching, leading to usually fine-grained soils (Dijkema, 1987b, Mueller et al., 2019, Oost et al., 2021). Despite their artificial origin, the man-made foreland-type salt marshes are appreciated for their value for nature conservation. Natural foreland salt marshes are relatively rare, although new natural salt marshes are developing in front of the sedimentation fields in Germany (Hartmann & Stock, 2019, see also section 2.8).
Figure 1. Photographic impression of the five characteristic salt marsh types of the Wadden Sea. Starting from the upper left: (i) back-barrier coarse-grained salt marsh in the lee of a dune ridge, (ii) green beach in the seaward area of a dune ridge, iii) a chenier-protected salt marsh, (iv) fine-grained foreland-type salt marsh mostly found on the mainland and largely man-made and (v) Hallig salt marsh that developed on a remnant part of the historic mainland, and which are not protected by a seawall. Photographs i, ii, and iv by Rijkswaterstaat (Joop van Houdt) and photographs iii and v by Martin Stock / LKN-SH.
Box 1: Geomorphology of a chenier island in the Wadden SeaChenier-protected salt marshes are located on chenier islands that differ from barrier islands in three ways (defined by Govers et al., 2021*, see Figure B1):
The following islands in the Wadden Sea are considered as Chenier islands in this QSR report (from west to east): NL: Richel, Griend, and Zuiderduintjes, LS: Lütje Hörn, Memmert, and Mellum. *In contrast to Govers et al. (2021) we classify Trischen a barrier island. Scharhörn has merged with Nigehörn, and this island complex now shows a closer resemblance to a barrier island. Additionally, we changed ‘intertidal mudflat’ to ‘intertidal flat’ in point 1 and added ‘next to sand’ to point 3. |
A Hallig is a salt-marsh island, which had been part of the mainland in historical times and became disconnected from the mainland by major flooding events. Hallig islands are not protected by a high seawall, but by smaller revetments (Figure 1). Hallig salt marshes accreted on low-lying old land and are mostly found in Germany. They show a greater resemblance with the fine-grained foreland salt marshes than with the coarse-grained barrier-connected salt marshes. Marshes located on islands that did not fit the description of a Hallig, barrier-connected, or a chenier-protected marsh were defined as 'other', for example, salt marshes on the island Langli in Denmark (Table 1 and Figure 2).
Summer polders may be flooded by seawater during extreme storm tides. Strictly speaking, and contradictory to the WSP (CWSS, 2010), most summer polders cannot be considered as salt marsh, because flooding by seawater is too infrequent. Consequently, the area of halophytic vegetation in summer polders is very limited. Because summer polders have a high potential for salt-marsh restoration (see, e.g., Koppenaal et al., 2022), their 2,200 ha were added as an extra category to Table 1.
Table 1. Recent extent (ha) of salt marshes and summer polders per sector specified according to their geomorphology. The pioneer zone has been defined as the area where vegetation covers ≥ 5%; in Schleswig-Holstein, this threshold value was 10%. Estuarine marshes are included with the mainland salt marshes. On the islands, de-embanked summer polders were added to the barrier-connected marshes; on the mainland to the foreland-type salt marshes. Governance sectors of the Wadden Sea, in which the salt marsh monitoring is carried out: NL = the Netherlands, LS = Lower Saxony, HH = Hamburg, SH = Schleswig-Holstein, DK = Denmark. See Annex I for methodology. The reported extent of salt marshes is based on vegetation and habitat mapping using the TMAP typology (Petersen et al., 2014).
About 57% of the current salt marshes in the Wadden Sea belong to the foreland-type salt marshes on the mainland (Table 1, Figure 2). These mainland marshes are largely man-made, developed within sedimentation fields, and constructed for coastal protection, agricultural exploitation, and land claim. During the last quarter of the last century the shift to nature conservation led to a change in management that varies today from a general minimum intervention to a strategic upkeep of parts of both the drainage and the groyne system. The scenery of the marshes today still resembles much that of a historical-cultural landscape by the imprints from the past, such as the geometric pattern of the marshes, remnants of extensive artificial drainage networks, and the brushwood groynes surrounding the sedimentation fields. At several sites, natural marshes are now developing in front of the man-made foreland marshes, a process which is described in detail in section 2.8. In order to enhance natural dynamics, various restoration measures have been carried out during the past decades, such as filling up parts of the drainage system and lowering the surface elevation by top-soil removal. These restoration efforts are summarised in section 2.7. To assist the decision making in policy and management, and to evaluate the status of the man-made foreland salt marshes, a conceptual reference model has been constructed (van Wesenbeeck et al., 2014) and was presented in the previous QSR (Esselink et al., 2017). Recently, civil engineering projects have been carried out to increase salt marsh area along the Dutch mainland coast. These will be described in more detail in section 2.9.
2.2 Salt marsh trends and distribution
The distribution of the salt marshes shows distinct variation across the Wadden Sea (Figure 2). Barrier-connected salt marshes on the mainland coast are restricted to the northern part of the Wadden Sea. Hallig salt marshes are almost entirely restricted to Schleswig-Holstein, except the Punt van Reide salt marsh in the Netherlands.
Since the first survey of 1995/2001, salt marshes showed a remarkable net increase of approximately 6,000 ha in twenty years’ time (Figure 3). The size of barrier-connected salt marshes on the islands increased across all sectors, and these marshes accounted for about half of the total increase.
The trend of increasing salt marshes on the islands differs clearly from the development of both mainland and Hallig salt marshes (Figure 3). Hallig salt marshes are almost completely enclosed by revetments (Figure 1), and their size therefore remains almost unchanged with a total extent of 2,230 ha (Table 1). Mainland marshes showed different trends among sectors.
In Lower Saxony and Denmark, overall net changes seem negligible. The small increase observed in Lower Saxony is partially based on data shifts. Specifically, the previous survey (2008/2014) did not include new area-wide data from Lower Saxony, and the current data compilation now includes approximately 400 ha of salt marshes in the estuaries along the rivers Ems and Elbe (Annex II), which were not covered in the two earlier surveys (Figure 3). Since the first survey, mainland marshes in the Netherlands increased with 620 ha (14%) and in Schleswig-Holstein with 2,460 ha (almost 30%; Figure 3).
Figure 2. Overview of the total area of salt marshes and summer polders in different parts of the Wadden Sea (governance sectors, see Table 1) specified according to their morphology. NL = the Netherlands, LS = Lower Saxony, HH = Hamburg, SH = Schleswig-Holstein, DK = Denmark. Same data as Table 1.
Figure 3. Development of the areal extent of salt marshes in different parts of the Wadden Sea based on four successive surveys; from left to right: 1995/2001, 2002/2007, 2008/2014, and 2015/2021. Data of the first three surveys are from Esselink et al. (2017). NL = the Netherlands, LS = Lower Saxony, HH = Hamburg, SH = Schleswig-Holstein, DK = Denmark.
2.3 Salt marsh zones and vegetation composition
In the 2009 and 2017 QSRs, the ageing of salt marshes, i.e. the extension of late-succession salt-marsh communities, at the expense of young-succession stages, was identified as the dominant process in most Wadden Sea salt marshes and led to an increased incidence of late-succession vegetation types, especially vegetation of Elymus athericus (Esselink et al., 2009; 2017; Bakker, 2014; Rupprecht et al., 2015). For example, the two largest back-barrier marshes in the Netherlands, each exceeding 1,000 ha (Terschelling and Schiermonnikoog), both feature a dominance of E. athericus (Annex II). In contrast, recent observations in both the Netherlands and Schleswig-Holstein indicate new development of salt marshes along the mainland coast, characterised by an increase of pioneer and/or low-marsh vegetation types (Figure 4). However, despite an increase in marsh area, the extent of pioneer and low-marsh vegetation types remained relatively constant on the Wadden Sea island marshes and on the mainland marshes of Lower Saxony, due to a continued succession from low-marsh to high-marsh vegetation. In Denmark, vegetation mapping has been conducted at a less detailed level, focusing on EU habitat types, thereby limiting the assessment of the succession stages of Danish salt marshes based on current maps.
In the mainland salt marshes of the Netherlands, the extent of high-marsh vegetation remained relatively constant: 1,220 ha in 2001 versus 1,290 ha in the most recent survey (Figure 4). The larger part of these marshes is grazed, which restrains the succession from low-marsh to high-marsh vegetation (Esselink et al., 2009; 2017). Since 2012, grazing has been re-introduced in parts of the mainland marshes of the Groningen coast to reduce the cover of E. athericus vegetation. A reduction of 7-8% was determined between 2008 and 2014 (Elschot et al., 2020), but it remained relatively stable thereafter. Approximately 25 percent of the marsh area (H1330) was still dominated by this late-succession type in 2020 (Annex II).
In the framework of salt marsh engineering, Spartina anglica was introduced and planted at several sites in the Wadden Sea during the 1920s until the 1950s (van Eerde, 1942; Esselink, 1998; Reise, 1994; Nehring & Hesse, 2008). It cannot be excluded that the planting material also included the sterile bastard Spartina townsendii, which would explain why S. townsendii is also found in the area today (Granse et al., 2021). S. anglica is the dominant and characteristic species of the S. anglica vegetation type in the TMAP vegetation typology (Petersen et al., 2014) and of habitat type H1320 (Spartina swards; Doody, 2008). S. anglica vegetation can now be found in almost every salt marsh of the Wadden Sea (Annex II), and the introduction of the species brought a great change in the Wadden Sea salt marshes. Cover of S. anglica is high in the German sectors, especially in Lower Saxony and Schleswig-Holstein, where nearly half of the pioneer marsh is formed by Spartina swards (Annex II). In the other sectors, the cover is much lower.
Figure 4. Development of the spatial extent of the different vegetation zones based on four consecutive salt marsh surveys: 1995/2001, 2002/07, 2008/2014, and 2015/2021 (from left to right) on the (A) Wadden Sea islands, (B) mainland coast, and (C) Halligen. Data of first three surveys are from Bakker et al. (2005) and Esselink et al. (2009; 2017). For graphical reasons, the salt-marsh areas of Hamburg (HH) have been pooled with Lower Saxony (LS); Hallig-type salt marshes in the Netherlands (NL) have been included in the mainland marshes. The pioneer zone is defined as the area where pioneer vegetation covers ≥ 5% (Petersen et al., 2014); in Schleswig-Holstein (SH), this threshold value is 10%. Marsh zonation was based on the TMAP vegetation typology (Petersen et al., 2014). In Denmark (DK), vegetation mapping was carried out at the more general level of EU habitat types and apart from the pioneer marsh, a further differentiation according to marsh zonation was not possible here.
2.4 Artificial drainage of salt marshes
To enhance marsh development in man-made foreland-type salt marshes, regular maintenance of the artificial drainage system was traditionally implemented (Esselink et al., 2009; 2017). With the recognition of conservation values of salt marshes in the 1970s and 1980s, the maintenance of artificial drainage systems was stopped completely over extensive areas to increase the natural dynamics of man-made salt marshes (WSP Target). Compared to previous QSRs (Esselink et al., 2009; 2017), the extent of recent drainage practices basically remained unchanged for all sectors and measures were mostly limited to maintenance and refurbishing of main channels.
In the Netherlands, maintenance of the artificial drainage system ceased in the eastern Dollard salt marshes in 1984 and in most other mainland marshes during the 1990s (Esselink, 2000; Dijkema et al., 2001). Field and collector drains are still regularly maintained in most privately owned marshes to facilitate livestock grazing. Along the coast in Friesland, the main channels are still regularly maintained. Re-profiling of both deeply incised field drains and collector drains were once-only measures in the early 2010s along the Groningen coast, mainly to facilitate safety for livestock. On the Dutch Wadden Sea islands, most back-barrier marshes are naturally drained, except for some older grazed marshes, where drainage measures have been carried out in the past in parts of the marsh near the dunes.
In Germany, the decrease in maintenance of the artificial drainage system was stimulated by the establishment of the national parks in the second half of the 1980s (Hofstede & Schirmacher, 1996).
In the Lower Saxon National Park, the marsh area with intervention in the drainage system decreased to around 10-15% during the last 10 years. Around 50% has never been drained and 35-40% has not been maintained in 20 years (Nationalparkverwaltung Niedersächsisches Wattenmeer, 2022). Recently, several projects have been carried out to restore salt-marsh hydrology and morphology to a more natural state, see section 2.7 for more detail. Restoration efforts towards more natural drainage were carried out in around 300 ha of salt marshes located both on the islands and along the mainland coast within the national park.
In Schleswig-Holstein, the border of the national park begins 150 metre further seaward than in Lower Saxony (Figure 5 – Transition between zone A and B). Within zone A, the traditional drainage system with field drains leading to collector drains and main channels is regularly maintained by the coastal protection agency. Some of the main channels in zone B are maintained when the functioning of the drainage in zone A is hampered. The area of intensively drained salt marshes on the mainland coast of Schleswig-Holstein remained constant over the last years and will remain constant under the present management regime. Zone C depicts the areas of near-natural or natural salt marshes without artificial drainage structures and is constantly growing (see section 2.8). Here, the main channels start to meander, but the drainage system as a whole does not reflect a completely natural creek system due to historical and partially continued drainage management in the landward part of the catchment area. In the salt marshes of the Hamburg national park, the drainage system has not been maintained since the 1950s.
In Denmark, drainage measures were cancelled at the beginning of this century. Only along the foot of both the Ribe Dike and the Ballum Dike parallel drains have been maintained on a regular base. In some salt marshes along the Ho Bay and near the mouth of river Varde Å, the drainage regime has been reduced over the last decades in order to rewet these marshes and increase their suitability as breeding habitat for meadow birds.
Figure 5. Drainage management of salt marshes in front of seawalls in Schleswig-Holstein. On the left side, managed drainage structures of field drains in 150-metre wide zone fringing the seawall, collector drains and main channels of two catchment areas are highlighted in blue as an example.
2.5 Vegetation management
During vegetation surveys, management practices such as livestock grazing, mowing, and minimum intervention are defined and have been recorded in the TMAP surveys. Grazing management was categorised in three classes of intensity defined on the basis of vegetation structure (Figure 6, Bakker et al., 2005): ‘intensive grazing’ indicated by a uniform short sward, ‘moderate grazing’ indicated by a heterogeneous sward with short and tall canopies, and ‘moderate/high’ when the intensity could not be determined.
In the Netherlands almost all mainland marshes are being grazed and the area with minimum intervention decreased since the previous survey of 2008/2014 (Figure 6). The limited availability of data on grazing management made it difficult to distinguish between high or moderate grazing intensity. On the islands, the area of minimum intervention remained more or less unchanged.
In Germany, salt marsh areas with minimum intervention increased since 1995 at the expense of livestock grazing, in Lower Saxony as well as in Schleswig-Holstein (Figure 6B). The most profound change in vegetation management in German salt marshes occurred in the second half of the 1980s. With the foundation of the national parks, livestock grazing was discontinued over extensive areas. For instance, ten years before TMAP monitoring started in 1995, only 3% of the mainland salt marshes in Schleswig-Holstein had a management of minimum intervention, and 90% was used for high-intensity livestock grazing (Esselink et al., 2009). These figures are in sharp contrast with the current situation where minimum intervention extends over 51% of these marshes, and the area of high-intensity grazing has dropped to less than 40% (Figure 6B).
In Denmark, the area with minimum-intervention management seemed to have doubled on both the island and mainland marshes between the first two surveys, but in the two most recent surveys, it decreased again. The survey methods varied from aerial livestock counts during the first survey to more recent assessment of the grazing status carried out through fieldwork for the EU habitat surveys (see Annex I). Data in Denmark may therefore not be fully comparable between years. If the results of the 2002/2007 survey are considered to be an outlier, then we may conclude that the marsh area with minimum intervention increased over time.
At the trilateral level, there are different views on the necessity of vegetation management in salt marshes (Bakker et al.,1997; Stock, 1997), and the fundamental principle of salt marsh management for nature conservation differs among countries. In the German national parks, the primary objective is to allow natural processes to continue with minimal intervention. In the Netherlands and Denmark, nature-conservation-management objectives focus more on using livestock grazing as a management tool to halt vegetation succession. Livestock grazing is advocated in these countries, especially in the semi-natural foreland salt marshes. On the scale of the entire Wadden Sea salt marshes, several researchers advise large-scale mosaics of different management regimes to conserve a habitat-specific vegetation and species diversity (de Vlas et al., 2013; Nolte, 2014; Wanner et al., 2014; van Klink, 2014; Stock & Maier, 2016; van Klink et al., 2016).
Figure 6. Vegetation management in the Wadden Sea salt marshes during a 20-year period based on four successive surveys: 1995/2001, 2002/07, 2008/2014 and 2015/2021 (from left to right) on the (A) Wadden Sea islands, (B) mainland coast and (C) Halligen. Livestock grazing includes both agricultural exploitation and livestock grazing as management tool for nature conservation. For graphical reasons, salt marshes of Hamburg (HH) have been pooled with Lower Saxony (LS), and Hallig-type salt marshes in the Netherlands (NL) with the mainland marshes. SH: Schleswig-Holstein; DK: Denmark.
2.6 Surface-elevation change and sea-level rise
Coastal salt marshes are potentially vulnerable to global warming and the associated accelerated levels of sea-level rise (SLR) (Bakker et al., 2016; Kirwan et al., 2016; Crosby et al., 2016). To evaluate the status of the Wadden Sea salt marshes in relation to SLR, an inventory of published and unpublished accretion studies in Wadden Sea salt marshes was carried out in the previous QSR (Esselink et al., 2017) and is updated below. The mean high tide (MHT) level forms approximately the lower boundary of salt marshes and changes in MHT have a greater effect on a salt marsh than similar changes in mean sea level. Therefore, the increase of local MHT was used as a parameter for SLR. Over the last century, increase in MHT varied within the Wadden Sea due to differences in vertical land movement and changes in wind climate (Bossinade et al., 1993; Philippart et al., 2024). Since 1900, the increase in MHT in the Wadden Sea has been in the order of 2.0-3.0 mm/yr, with the highest values observed in the German sector east of the Elbe (Wahl et al., 2011; Jensen et al., 2014; Oost et al., 2017).
Most accretion studies were relatively recent and, to compare the surface-elevation changes (SEC) from about the same period, data from before 2000 were disregarded. The survey yielded data from 38 different salt marsh sites, and two summer polders (Annex III). SECs clearly differed between different types of salt marsh in the Wadden Sea (Figure 7). The highest mean SECs were found in foreland salt marshes with, on average, values at least twice as high as in back-barrier and chenier-protected marshes (8.7 mm/yr versus 3.5 and 2.1 mm/yr). Marsh resilience to SLR may be underestimated by SEC. Firstly, salt marshes feature a feedback mechanism between surface elevation in the tidal frame, inundation frequency and sedimentation which results in increased sedimentation rates at lower elevation (French, 1993). Secondly, spatial variation in SEC within a marsh complex (high in young and seaward parts versus low in old and back (landward) parts) may contribute to the resilience of a marsh (Bartholdy et al., 2010; Kirwan et al., 2016; Cornacchia et al., 2024). Thirdly, in back-barrier marshes overwash events during storms may deliver additional sediment onto the marsh surface (Schuerch et al., 2018). The capacity of barrier-connected and chenier-protected marshes to keep pace with an accelerated SLR due to global warming will nevertheless be limited due to their low SEC, especially compared to most foreland marshes with higher SECs (Figure 7). Recent studies showed that in the Wadden Sea the annual availability of muddy sediments (key component for salt marshes to accrete) is limited. The main mud source is the marine North Sea Continental Flow which produces a fading sediment plume along the coast from the western end of the Wadden Sea up to Denmark causing a decrease in mud availability in the downdrift direction (Colina Alonso et al., 2024).
Hallig salt marshes show on average a lower SEC (1.6 mm/yr) than the local increase of MHT as the surrounding revetments or constructed low banks impede the flooding of these marshes. Hence, they have a low potential for sediment import and livestock can further reduce SEC (Schindler et al., 2014; Schulze et al., 2021).
Flooding frequency of summer polders is low (often less than once a year), leading to extremely low or negative SECs (Figure 7). Hence, summer polders face an increasing elevation deficit. This development may be reversed by restoration of tidal influences. In a de-embanked summer polder on the coast of Friesland for instance, SEC in the restored salt marsh exceeded the increase in MHT by more than 300% (Esselink et al., 2015; Koppenaal et al., 2022).
Figure 7. Surface-elevation change (SEC) in Wadden Sea salt marshes and summer polders. The graph is a compilation of both changes in surface elevation from levelling studies, Sedimentation Erosion Bar (SEB) measurements, and marker-horizon techniques including clay-layer thickness measurements (Nolte et al., 2013). Elevation changes are based on overlapping and varying periods in time, mostly starting around 2000 or a more recent date. The horizontal blue bar gives the range of the long-term increase of MHT level. Annex III presents the data used in the graph.
2.7 Salt marsh restoration efforts
In many salt marshes of the Wadden Sea, artificial geomorphology and hydrology persist despite abandonment of drainage management and changes in land use. In addition, tidal flooding has been reduced to very low levels by embankment of summer polders which today still cover 2,200 ha (Table 1). In the Netherlands, Lower Saxony and the island of Neuwerk (Hanseatic city of Hamburg), restoration projects have been carried out to restore natural processes such as tidal flooding, sedimentation, and erosion, and vegetation succession (Figure 8). Approaches for salt marsh restoration include: 1) opening/removal of summer-dikes to restore tidal flooding and sedimentation (de-embankment), 2) de-activation of the artificial drainage system to restore natural hydrology, and 3) lowering the surface elevation by top-soil removal to set-back vegetation succession and allow a more natural drainage system to develop (Figure 9).
Figure 8. Total size of salt-marsh restoration efforts on (A) the islands and (B) at the mainland coast of in the Netherlands (NL) and Lower Saxony with Hamburg (LS+HH) disaggregated over three main restoration types: de-embankment, de-activation of artificial drainage, and top-soil removal. * = With top-soil removal in part of the area.
Figure 9. Salt marsh restoration sites, examples of the three main restoration types: a) Langwarder Groden prior to and 4 years after de-embankment, b) Norderney Ostheller prior to and one year after de-activation of artificial drainage, c) Neuwapeler-Außengroden prior to and one year after top-soil removal (all Photographs by N. Hecker, NLPV).
Restoration efforts in the Netherlands have been focusing on de-embankments, with a total of about 600 ha divided over 10 different sites. In Lower Saxony, nearly 1,000 ha of salt marshes at 17 sites have been restored (Figure 8, Annex IV). Initial habitat conditions at restoration sites can be highly variable and salt marsh development after restoration works differ accordingly (Rupprecht et al., 2023). Hence, a synthesis on the achievement of restoration targets over all restoration sites is challenging.
Figure 10. Habitat development at a selection of salt-marsh restoration sites in Lower Saxony prior to and after restoration measures in three restoration approaches. De-embankment: a) Hauener Hooge (monitoring period: 22 years) and b) Langwarder Groden (monitoring period: 5 years). Deactivation of artificial drainage: c) Leybucht-Mittelplate (monitoring period: 5 years), d) Norderney Ostheller (monitoring period: 2 years (construction part I, 28 ha) and 9 years (construction part II, 53 ha) respectively). Top-soil removal: e) Neuwapeler-Außengroden (monitoring period: 3 years). Marsh zones are according to the TMAP-typology (Petersen et al., 2014).
In case of de-embankment, most sites were originally dominated by fresh grassland. After de-embankment, high- and low-marsh vegetation established and increased in cover (Figure 10a and b). Size of the de-embanked site, initial surface elevation, surface relief, and drainage system all affect the development of salt-marsh vegetation after de-embankment (Wolters et al., 2005; Brooks et al., 2015; Chang et al., 2016; Rupprecht et al., 2023).
When deactivation of the artificial drainage system was applied, the sites were usually dominated by high-marsh vegetation prior to restoration works. Results from the restoration sites show that high-marsh vegetation is still dominant (Figure 10c and d). Filling of the drainage system with local sediment required top-soil removal and reallocation of sediment in a small number of the restoration sites. At Norderney Ostheller (Figure 10d) the area of top-soil removal is three times larger than at Leybucht Mittelplate, resulting in a greater reduction of high marsh cover. Successful deactivation of field and collector drains along with subsequent rewetting in these restoration sites, depend critically on considering site-specific hydraulic conditions. This approach ensured an increase in both the duration of flooding and the flooded area (Rupprecht et al., 2023).
Restoration sites with top-soil removal generally started with a high-marsh vegetation and had low-marsh vegetation establishing within three years after completion of restoration works (Figure 10e). After top-soil removal, access of the tidal water to the restoration site to (re-)initiate natural processes such as tidal flooding, sedimentation and erosion, and the creation of a soil surface with shallow slope proved essential for the development of creeks and a more diverse geomorphology of the marsh platform (Rupprecht et al., 2023).
In general, local drainage structures, hydraulic conditions, surface relief, and surface elevation in relation to mean high tide seem to be critical parameters for the successful planning and realisation of salt-marsh restoration efforts, as they are important drivers of vegetation development after restoration efforts were taken. This seems to be in line with results in previous studies (e.g., Wolters et al., 2005; Brooks et al., 2015). Wolters et al. (2005) show that restoration success, defined by the number of target plant species expressed as a percentage of the regional species pool, was higher in larger restoration sites (>100 ha) with larger elevation gradients. Furthermore, young (< 20 years) restoration sites contained more target plant species than older sites. Some results shown in this section were collected only a few years after the restoration measure was completed (Figure 10e) and the effects on the vegetation composition may not have stabilised yet. To achieve the overarching aim of a more natural-like salt marsh, a combination of different restoration methods (e.g., de-embankment and top-soil removal) may be beneficial.
2.8 Natural expansion of foreland-type marshes
At several sites in Germany, new foreland salt marshes developed naturally, i.e. without human interference by civil engineering measures (Hartman & Stock, 2019, Figure 11). In Lower Saxony, more natural salt marshes are developing at the seaward fringe of foreland salt marshes in the two large bights of the mainland coast: Jadebusen and Leybucht, although in the latter case extra shelter was provided to the Bay by both coastal protection (the construction of Speicherbecken Leyhörn) and an offshore drilling rig with a service road on the intertidal flats. In Schleswig-Holstein, over 50% of the foreland salt marsh expansion since 1988 took place under minimum intervention, while other parts of the expansion happened under active ditching and draining by the coastal protection agency.
One example of natural salt marsh development can be found in the Dieksanderkoog area, close to the mouth of the Elbe estuary in southern Schleswig-Holstein (Figure 11). Since the establishment of the national park in 1985, grazing has been reduced or abandoned, followed by cessation of both ditching and upkeep of brushwood groynes.
Figure 11. Development of salt marsh areas between 1980 and 2021 at Dieksanderkoog, Schleswig-Holstein. The hatched areas in the right image are grazed salt marshes. The dotted line in the right picture divides the older anthropogenic marshes from the newly formed natural marshes. The remainder of the outer brushwood groynes, which have not been maintained since the late 1990s, are on the dotted line. The vegetation types are based on the TMAP typology (Petersen et al., 2014).
Between 1980 and 2021, around 400 hectares of natural salt marshes have developed (Figure 11, Hartman & Stock, 2019). The pioneer marshes near the mudflat have doubled in size since 1980. Between 1988 and 2001, the natural marsh was exclusively composed of Salicornia and Spartina vegetation types, after which a mosaic of types from all salt marsh zones began to form.
Currently, Dieksanderkoog encompasses the biggest coherent naturally formed foreland salt marsh in the Wadden Sea and can be regarded as a reference for natural salt marsh dynamics (Van Wesenbeeck et al., 2014; Esselink et al., 2017). In contrast to the drainage systems in anthropogenic salt marshes, the naturally formed salt marsh areas have meandering creeks and a fourfold lower length per area ratio, compared to the neighbouring anthropogenic salt marshes (Hartmann & Stock, 2019). This allows the naturally formed salt marsh to retain more water and to develop a natural hydrology (Hartman & Stock, 2019).
2.9 Construction of salt marshes
In the past decade, awareness has been growing among policy makers and government agencies that coastal marshes can form an integral part of nature-based solutions while dealing with societal and economic issues, e.g., as part of coastal protection (Temmerman et al., 2013). Plans to build new brushwood groynes to stimulate marsh development are currently considered on several locations in the Dutch part of the Wadden Sea, among others in the Ems estuary and east of the Lauwersmeer polder.
Brushwood groynes alone are not always enough for the creation of salt marshes. At sites with high hydrodynamic energy and a bed level that is too low for vegetation growth, civil engineering approaches have been tested to create or expand salt marshes. An example is the building of a new salt marsh close to the city of Delfzijl, in the Ems estuary (Baptist et al., 2021).
Through civil engineering, salt marshes can be constructed in locations unsuitable for natural marsh development. However, using this approach also poses additional concerns. Artificially developing salt marshes conflicts with targets to achieve an increased area of salt marshes with natural morphology and dynamics. Moreover, it can replace other protected and important habitat types, such as bare intertidal flats that provide important feeding habitats for migratory birds (Kleefstra et al., 2022). Finally, it could prevent natural salt-marsh development at sites where this is expected in future. In conclusion, the renewed interest in constructing salt marshes needs a careful approach.
3. Assessment
3.1 Introduction / Summary
The general trilateral aim for salt marshes is an adequate conservation of the full range of variety of salt marshes to allow natural processes to operate within this habitat, with special emphasis on flora and fauna and by this to maintain or restore a favourable conservation status. To reach this aim, the following five targets for salt marshes have been agreed upon in the Wadden Sea Plan (CWSS, 2010):
- To maintain the full range of variety of salt marshes typical for the Wadden Sea landscape;
- To achieve an increased area of salt marshes with natural dynamics;
- To achieve an increased area of natural morphology and dynamics, including natural drainage of mainland salt marshes, under the condition that the present surface area is not reduced;
- To maintain a salt-marsh vegetation diversity reflecting the geomorphological conditions of the habitat with variation in vegetation structure;
- To maintain or to achieve favourable conditions for all typical species.
3.2 To maintain the full range of variety of salt marshes
Target evaluation
From a morphological view, at least five types of salt marsh may be distinguished in the Wadden Sea, viz.: (a) back-barrier salt marshes, (b) green beaches (c) chenier-protected marshes, d) foreland-type marshes and (e) Hallig salt marshes (section 2.1). These categories are related to the geomorphology of the Wadden Sea landscape and should therefore be considered as landform types. The harmonised TMAP salt marsh surveys, however, cover vegetation and habitat types only and do not include geomorphology. Mapping of landform types was recommended in the 2009 and 2017 QSRs (Esselink et al., 2009; 2017). This recommendation has not been fully implemented yet, which prevents a quantitative evaluation of this target. For practical reasons, salt marsh habitats of green beaches were merged, with back-barrier salt marshes, into the group of barrier-connected salt marshes.
Summer polders should not be considered as salt marsh, because the flooding by seawater is generally too infrequent for halophytic vegetation to develop. De-embankment of summer polders, however, may specifically contribute to the restoration of wide salt marshes.
Conclusions
Range of salt marsh per geomorphological type.
- Barrier-connected salt marshes make up about 40% of the salt marshes in the Wadden Sea. From a geomorphological point of view, these salt marshes can largely be classified as natural salt marshes. Since the first TMAP survey in 1995/2001, these salt marshes showed a remarkable Wadden Sea wide net increase.
- Chenier-protected marshes are now added as a separate geomorphological salt-marsh type, and they cover about 6% of the salt marshes found on islands.
- Foreland-type salt marshes on the mainland make up more than 50% of the salt-marsh area in the Wadden Sea. The present salt marshes are largely man-made (section 2.1). In the Wadden Sea, enhancement of salt marsh development goes back to at least the 16th century and man-made salt marshes therefore also form a cultural-historical entity of the Wadden Sea landscape.
- The size of the foreland salt marshes is generally dependent on the upkeep of the brushwood groynes at the seaward marsh edge. Natural expansion without human intervention is the exception and is mainly confined to the Schleswig-Holstein sector and to the Leybucht and Jadebusen in Lower Saxony (see also section 2.8).
- The area of Hallig marshes has remained the same as they are enclosed by revetments.
Accretion rates and sea-level rise
- Barrier-connected salt marshes have relatively low accretion rates: on average only slightly higher than current sea-level rise. This does not imply that sea level rise forms a threat to these salt marshes on the short term, since there are various mechanisms for salt marshes to adapt to sea-level rise. On the long term, however, because of low levels of surface elevation change (SEC) these marshes may not be able to keep pace with an accelerated rate of sea-level rise.
- Chenier-protected salt marshes feature an ephemeral nature (Box 1). At this moment, it seems impossible to forecast the impact of potential changes in storm climate as a result of global warming on these marshes.
- Foreland-type salt marshes feature a wide range in accretion rates. They are able to keep up with current sea-level rise and are more resilient to an accelerated SLR.
- Hallig salt marshes are generally spatially fixed by revetments. In most cases, accretion rates are lower than the current local rise in sea level. An accelerated SLR is therefore considered as major future threat to Hallig salt marshes.
- Summer polders are inundated rarely and therefore receive low amounts of sediment and consequently feature a very low SEC (Figure 7). Vertical accretion may successfully be restored by de-embankment or coastal realignment.
3.3 To achieve an increased area of salt marshes with natural dynamics
Target evaluation
This target aims to increase the size of natural salt marshes (CWSS, 2010), where both geomorphology and vegetation are free of any human intervention. Natural processes are still in place and have been so since the salt marshes started to develop. Most of the target evaluation in the 2009 and 2017 QSRs is still valid (Esselink et al., 2009; 2017).
In line with the preceding QSRs, natural expansion of salt marshes has continued with a natural expansion of the barrier-connected island salt marshes and foreland salt marshes in Schleswig-Holstein. The total salt-marsh area in the Wadden Sea increased by roughly 6,000 ha since the first trilateral survey of 1995/2001.
The larger part of the barrier-connected salt marshes is located on the so-called island tails of the islands west of the Elbe estuary. These islands undergo a bio-geomorphic succession (de Groot et al., 2016). The construction of an artificial dune ridge on some islands accelerated this succession. To restore an affected island tail to its original state may be impossible, because large-scale bio-geomorphic processes are dominant. De Groot et al. (2016) recommend being cautious with interventions. Only in case when bottlenecks for pioneer species are perceived, do these authors advise to restore actively hydrodynamic and aeolian processes locally to set back vegetation succession.
Conclusions
- In the Wadden Sea, natural salt marshes are mainly found as coarse-grained barrier-connected, chenier-protected (both island) and some foreland-type salt marshes. At various sites, the size of barrier-connected salt marshes is greater today than during historical times due to the construction of artificial dune ridges.
- The former construction of artificial dune ridges did not only facilitate the extension of barrier-connected salt marshes, but also resulted in reduced spatial dynamics and an accelerated vegetation succession of these salt marshes.
- Re-introduction or restoration of geomorphological processes may locally result in a greater spatial and temporal variation in barrier-connected salt marshes.
- The naturalness of barrier-connected salt marshes that have been disturbed by artificial drainage may be improved by filling up drains and excavating former creeks and depressions.
- Natural foreland salt marshes are relatively rare and found almost only in Schleswig-Holstein as well as in the Leybucht and Jadebusen in Lower Saxony (section 2.8). Artificial or man-made foreland salt marshes cannot be restored to natural salt marshes, which will be discussed in more detail in the next target.
3.4 To achieve an increased natural morphology and dynamics, including natural drainage of mainland salt marshes, under the condition that the present surface area is not reduced
Target evaluation
To induce a more natural morphology of the artificial foreland salt marshes, maintenance of the artificial drainage system was discontinued in over 70% of the area and this remained relatively the same since the last QSR (Esselink et al., 2017). As a result, salt marshes slowly develop towards a state that features elements and processes that are more natural such as the development of depressions and the build-up of levees (Esselink et al., 1998). When the artificial drainage is no longer maintained this leads to rewetting of the salt marsh, which can retard vegetation succession (Esselink et al., 2009).
After abandonment, part of the artificial drainage system is silted up relatively quickly, but the straight pattern of the drainage system is generally very resilient (van Wesenbeeck et al., 2014). A natural system with meandering creeks normally starts to develop in a very early stage of salt marsh development (Esselink et al., 2017 and references therein). Once a salt marsh has been formed, however, creeks remain relatively stable and straight drains will not easily evolve into meandering creeks (van Duin & Dijkema, 2003). Meandering creeks may develop in depressions, and also in expanding marshes, in the transition zone from pioneer marsh to the intertidal flat.
An additional strategy to diversify the morphology of artificial salt marshes is to increase their width and restore wide marshes with a complete hydrodynamic gradient. The great majority of the present mainland salt marshes form only a narrow fringe along the coast. Consequently, in only few situations, these salt marshes feature the complete hydrodynamic gradient of natural wide salt marshes. De-embankment of summer polders will specifically contribute to the restoration of wide marshes.
Natural salt marshes are generally spatially dynamic: they either expand or erode. Most of the existing foreland salt marshes, however, are depending on brushwood groynes for protection against erosion (e.g., Dijkema et al., 2001). In practice, this implies that their seaward boundary has become fixed. Restoration of spatial dynamics would require an adaptive long-term management of the brushwood groynes. A temporary phase of neglect and erosion might induce space where new pioneer salt marshes can develop once the groynes are reconstructed again.
Conclusions
- The target has been realised to a considerable extent. By ceasing the maintenance of the artificial drainage, artificial salt marshes slowly develop a more natural morphology.
- The straight pattern of the artificial drainage is expected to be very resilient. Development of meandering creeks is confined to salt marsh depressions and to the transition zone from pioneer marsh to the intertidal flats.
- Most existing mainland salt marshes are relatively narrow. De-embankment of summer polders may contribute to the restoration of wide salt marshes, which feature a greater internal hydrodynamic gradient.
- In comparison with natural salt marshes, artificial salt marshes lack spatial dynamics through fixation of the seaward edge by the brushwood groynes. An adaptive management of the groynes is required to regulate erosion and to create chances for new pioneer marsh to develop.
- Creating salt marshes by civil engineering conflicts with natural dynamics and potentially prevents natural marsh to develop.
3.5 To maintain a salt marsh vegetation diversity reflecting the geomorphological conditions of the habitat with variation in vegetation structure
Target evaluation
From the evaluation of the previous target, it follows that an improved natural morphology is a pre-requisite to achieve higher vegetation diversity in artificial salt marshes. In some artificial marshes, increased vegetation diversity has been observed as a result of change in management and an increased natural morphology. Whereas being a common process in most artificial marshes, ageing may be retarded or prevented in marshes with an improved morphology and/or an increased re-wetting of the marsh.
Barrier-connected salt marshes are also susceptible to ageing. In salt marshes that have been influenced by human intervention, such as the construction of an artificial dune ridge or artificial drainage, succession and dominance of Elymus athericus vegetation is enhanced. Natural dynamics, e.g., overwashes, may prevent, or at least hamper this succession.
On the trilateral level, a method for the evaluation and assessment of vegetation diversity still has to be developed. Therefore, an evaluation of the target cannot be given yet.
Conclusions
- An increased natural morphology is a pre-requisite in order to achieve higher vegetation diversity in artificial salt marshes.
- On the trilateral level, a method for the evaluation and assessment of vegetation diversity still has to be developed.
- In principle, there are two different views on the target and on how the target can be reached. The two views have been integrated in the Wadden Sea Plan (2010): 1) the prime objective is a vegetation development in relation to the geomorphological conditions with a minimum of anthropogenic influences, and 2) the main aim is to prevent dominance by species-poor vegetation of late successional stages, and to preserve and enhance vegetation diversity in a semi-natural landscape with livestock grazing as a management tool. In this approach vegetation diversity is thus the outcome of the interaction between geomorphological conditions and grazing.
3.6 To maintain favourable conditions for all typical species
Target evaluation
It may be questioned whether this target can be evaluated in a sound way. It will be clear that it is not feasible to assess all characteristic salt marsh species in a standardised programme. There are simply too many groups of organisms living in salt marshes, and hence, a selection of indicator or umbrella species or species group might be required. Furthermore, an evaluation method would have to be developed.
How to proceed with the evaluation of the target may also differ according to the prime objective for nature conservation. It should be recognised that species differ in their habitat requirements, and hence, to preserve favourable conditions for all typical species, a variety of salt marsh habitats is a prerequisite. If the management objective is “a vegetation development in relation to the geomorphological conditions with a minimal anthropogenic influence”, the different salt-marsh habitats present may be accepted as the natural variety of habitats.
If the management objective is to preserve and enhance vegetation diversity, and livestock is accepted as management tool, the variety of habitats may be enhanced. In this setting, large-scale mosaics of different management regimes may support the maintenance of the greatest possible habitat and species diversity (Table 2; Wanner et al., 2014; van Klink et al., 2016).
Conclusions
- It is not feasible to evaluate the target in a sound way. An evaluation method is lacking and would have to be developed first.
- Where applicable, a large-scale mosaic of different management regimes, including minimum intervention, may support the different habitat requirements of many plant, invertebrate and vertebrate species (Table 2).
Table 2. Overview of the diversity of salt marsh plants and animals in relation to the management regime in Noord-Friesland Buitendijks (Netherlands). Stocking density and rotational treatment refer to grazing by livestock. Darker shading indicates a higher number of species. (With geese and voles: darker shading indicates higher numbers of individuals). For birds and invertebrates, every regime has its own characteristic species. From a large-scale perspective, diversity can be maximised by using a spatial mosaic of different management regimes. The index values for passerines during winter are based on estimates. From: de Vlas et al. (2013).
4. Recommendations
4.1 Recommendations for monitoring and research
Most recommendations for monitoring and research from the 2009 and 2017 QSRs are still valid (some slightly revised):
- The monitoring according to the TMAP guidelines should be fully implemented with a time interval of six years in all sectors. In order to assess the “favourable conservation status” of the Habitat Directive on the trilateral level, the TMAP Expert Group Salt Marshes and Dunes (EG-SMD) concluded that the TMAP monitoring according to TMAP guidelines is a prerequisite.
- Monitoring of artificial drainage in salt marshes. The TMAP monitoring assessed the time since the last human intervention, but this did not give any information about the actual status of the drainage system. The EG-SMD recommends adapting the monitoring on drainage according to a new harmonised method.
- The TMAP monitoring should be extended with monitoring of marsh surface elevation change (SEC) in relation to MHT. SEC is an essential parameter for the evaluation of salt-marsh development linked to accelerated rates of sea-level rise in times of climate change. Monitoring of SEC on selected permanent transects or monitor stations is therefore strongly recommended.
- A method to evaluate and assess the target “to maintain a salt-marsh vegetation diversity reflecting the geomorphological conditions of the habitat with variation in vegetation structure” is lacking and should be developed on the basis of the TMAP typology.
- Investigate and harmonise existing plant species monitoring data available in the Wadden Sea. The TMAP monitoring programme does not provide monitoring data at the level of plant species. In order to assess processes of salt marsh change as well as the colonisation of new plant species, monitoring of plant species composition in permanent plots is required. This data is currently obtained in some, but not all sectors (Annex I). Ideally, this monitoring should be executed in relation with marsh elevation and management. For the next QSR we recommend including a section analysing the vegetation change in permanent plots, with the purpose of answering the target “To maintain a salt-marsh vegetation diversity reflecting the geomorphological conditions of the habitat with variation in vegetation structure”.
- Distinction of secondary pioneer vegetation. The primary pioneer zone and the secondary pioneer plant communities in the inner salt marsh are not ecologically equivalent. To assess both communities separately, it is advised to subdivide the following two TMAP vegetation types: the Spartina anglica type (S.1.1) and the Salicornia spp. / Suaeda maritima type (S.1.2; Petersen et al., 2014).
- Develop a standardised geodata format about 1) landform type, 2) legal protection status, 3) ownership and if possible 4) land use. To analyse vegetation maps or vegetation changes, data on landform type as well as legal protection status are relevant. So far, such information is not available at the trilateral level.
- Continuation of long-term study sites and incorporation of these sites into, e.g., the International Long-Term Ecological Research sites (ILTER).
New recommendations:
- Estuarine marshes should be identified as a separate landform type as part of the foreland-type marshes on a geomorphological level. Estuarine marshes differ from other foreland-type marshes in salinity and vegetation composition and should be assessed as a different landform in the next QSR. Distinction parameters, e.g., salinity, need to be formulated.
- Re-evaluate target: “To achieve an increased natural morphology and dynamics, including natural drainage of mainland salt marshes, under the condition that the present surface area is not reduced”. Erosion is part of the natural dynamics of salt marshes. Therefore, this target needs to be re-evaluated to ensure that it does not conflict with target “To achieve an increased area of salt marshes with natural dynamics”.
- Compile an overview of available data on carbon sequestration related to different marsh types. Recently, UNESCO published a study showing the importance of Wadden Sea marshes as blue carbon sinks (Duarte et al., 2021). To advance this topic, the EG-SMD recommends that an overview of the available data on carbon sequestration is made.
- The Surface-Elevation Change varies widely between seemingly comparable marshes and further research is recommended.
4.2 Recommendations for management
These recommendations from the 2009 and 2017 QSRs are still valid:
- At island salt marshes that have been previously affected by the construction of artificial dune ridges or sand dikes, resulting in the loss of specific habitat types, a setback of vegetation succession can be pursued by restoring hydrodynamics and aeolian dynamics, e.g., by initiating a wash-over by removing parts of the sand dike; otherwise it is advised to refrain from intervention in order to let natural processes take over.
- The development of naturally protruding salt marshes is best guaranteed by leaving the geomorphology of both the growing marsh and the adjacent intertidal mudflats undisturbed. Cliff erosion should be considered as a natural process in both natural and artificial salt marshes and should not automatically be interrupted by countermeasures.
- Wide salt marshes have a higher conservation value than narrow marshes. De-embankment of summer polders may specifically contribute to the restoration and formation of wide salt marshes. Moreover, de-embankment contributes to the areal extension of the current salt marshes.
- In order to allow more natural creek systems to develop in artificial salt marshes, it is advised to refrain from any maintenance on the drainage system here. If it is considered necessary to prevent waterlogging at the foot of the seawall, the upkeep of the artificial drainage should be confined to this purpose only.
- There are two different approaches in conservation management of Wadden Sea salt marshes (§3.5). When the undisturbed course of natural processes is the main objective, a management of minimum intervention is applied. In case the aim is (an) enhancement of biodiversity, livestock grazing is usually used to control vegetation succession. At the scale of the Wadden Sea salt marshes, maximum biodiversity can be achieved by the application or continuation of a large-scale mosaic of different management regimes including a regime of minimum intervention. Therefore, we recommend maintaining both approaches.
- Salt marsh management is part of the cultural history of the Wadden landscape. Sites with an unfavourable conservation status and/or low potential for increased naturalness can be considered for restoration measures, such as top-soil removal, re-arrangement of the artificial drainage system, or even a re-design of the entire marsh area.
5. Summary
Salt marshes in the Wadden Sea extend more than 42,000 ha, which represents approximately 20% of the total of coastal salt marshes in Europe. Regarding their geomorphology, at least five types of salt marshes are distinguished in the Wadden Sea Area: (a) back-barrier marshes, (b) green beaches, (c) chenier-protected marshes, (d) foreland-type marshes, and (e) Hallig salt marshes. In this report, the status of salt marshes is primarily assessed based on vegetation and habitat, and less on geomorphology. In this sense, halophytic vegetation on green beaches has been merged with back-barrier salt marshes into the group of barrier-connected salt marshes. Sometimes, summer polders are mentioned as extra salt marsh type in the Wadden Sea. However, the vegetation can mostly not be qualified as salt marsh, since the influence of seawater is too limited. Future discussions will elaborate on the status of estuarine marshes.
Salt marshes continued their expansion and increased by about 6,000 ha since the 2004 QSR. This increase occurred in barrier-connected island salt marshes as well as in foreland-type mainland. Despite the recent extension of salt marshes, the extent of pioneer and low-marsh vegetation types remained relatively constant, whereas vegetation of late-succession stages increased, especially Elymus athericus vegetation. The latter has become the most dominant vegetation type in the high-marsh zone.
Foreland-type marshes on the mainland account for approximately 57% of the current salt marshes in the Wadden Sea. Natural foreland-type salt marshes are relatively rare, and the majority are man-made. Compared to the previous QSR, the extent of recent drainage practices remained mostly unchanged. Vegetation management through mowing or grazing by livestock mostly reduced in Germany, while it slightly increased in Denmark, and more profoundly in the Netherlands.
In order to evaluate the status of Wadden Sea salt marshes in relation to current sea-level rise, available data on surface-elevation changes (SEC) were collected. Foreland-type salt marshes showed the highest mean elevation increase of 8.7 mm/yr, i.e. well above the increase rate of Mean High Tide (MHT) (2.0-3.0 mm/yr). With an average value of 3.5 mm/yr, SEC in barrier-connected salt marshes just exceeded the increase of MHT levels. With a mean rate of 1.6 mm/yr, Hallig salt marshes lag behind MHT increase. For the Hallig marshes this may be (partially) explained by disturbance of sediment supply to these marshes by coastal protection measures. Summer dikes surrounding the summer polders provide an even stronger disturbance and surface-elevation changes become negligible.
One of the targets of the Wadden Sea Plan is to transform artificial salt marshes into more natural states. In Lower Saxony, restoration projects were launched in some artificial salt marshes where natural development had been prevented by previous anthropogenic impact. The area of foreland-type marshes has been increasing in recent decades, by de-embankments and more natural foreland-type marshes that develop in front of the sedimentation fields. Additionally, as part of the coastal protection marshes were created in the Netherlands by civil engineering, which on one hand increases the area of salt marshes but on the other hand may conflict with increasing natural dynamics and potentially preventing natural marsh development.
Many of the recommendations from the previous QSR are still valid. In addition, further harmonisation of the data is recommended in order to make full use of the spatial-data sets that are available in the framework of TMAP monitoring.
About the authorsK. Elschot1, P. Esselink2, J. Frikke3, K. Jensen4, N. Janinhoff-Verdaat5, M. Paarup Thomsen6, M. Padlat7, J.T. van der Wal1, W.E. van Duin8, M.E.B. van Puijenbroek1, F. Rupprecht9, M. Stock10 1 Wageningen University and Research, Ankerpark 27, 1781 AG Den Helder, NL 2 PUCCIMAR Ecological Research and Consultancy, Vries, NL 3 Nationalpark Vadehavet, Havnebyvej 154, 6792 Rømø, DK 4 Applied Plant Ecology, Universität Hamburg, Ohnhorststr. 18, 22609 Hamburg, DE 5 Lower Saxony Water Management, Coastal and Nature Protection Agency, Hannover, DE 6 Agency for Green Transition and Aquatic Environment, Jacob Gades Allé 12a, 6600 Vejen, DK 7 Landesbetrieb für Küstenschutz, Nationalpark und Meeresschutz Schleswig-Holstein, Schloßgarten 1, 25832 Tönning, DE 8Artemisia, Salt-marsh research, Graaf Willem II straat 258, 1785 KL Den Helder, NL 9 Wadden Sea National Park Authority of Lower Saxony, Virchowstr. 1, 26382 Wilhelmshaven, DE 10 Doerpstedt 5, 25873 Rantrum, DE |
References
Adam P. (1990) Saltmarsh ecology. Cambridge University Press, Cambridge. 461 pp.
Allen J.R.L., Pye K. (1992) Coastal saltmarshes: their nature and importance. In: J.R.L. Allen & K. Pye (eds). Saltmarshes. Morphodynamics, conservation and engineering significance. Cambridge University Press, Cambridge. p. 1–18.
Bakker J.P. (2014) Ecology of salt marshes. 40 years of research in the Wadden Sea. Wadden Academy, Leeuwarden. 99 pp.
Bakker J.P., Baas A.C.W., Bartholdy J., Jones L., Ruessink G., Temmerman S., van de Pol M. (2016) Environmental Impacts – Coastal Ecosystems. In: M. Quente & F. Colijn (eds). North Sea Region Climate Change Assessment. Springer Open. p. 275–314. doi 10.1007/978-3-319-39745-0_9.
Bakker J.P., Berg M.P., Grootjans A.P., Olff H., Schrama M., Reijers V.C., van der Heide T. (2023) Biogeomorphological aspects of a model barrier island and its surrounding – Interactions between abiotic conditions and biota shaping the tidal and terrestrial landscape: A synthesis. Ocean and Coastal Management 239: 106624.
Bakker J.P., Bunje J., Dijkema K.S., Frikke J., Kers B., Körber P., Kohlus J., Stock M. (2005) Salt marshes. In: K. Essink, C. Dettmann, H. Farke, K. Laursen, G. Lüerßen, H. Marencic & W. Wiersinga (eds). Wadden Sea Quality Status Report 2004, Wadden Sea Ecosystem No. 19, Trilateral Monitoring and Assessment Group, Common Wadden Sea Secretariat. Wilhelmshaven. p. 163–179.
Bakker J.P., Esselink P., Dijkema K.S. (1997) Salt-marsh management for nature conservation, the value of long-term experiments. Wadden Sea Newsletter 1997(1): 19–24.
Balke T., Stock M., Jensen K., Bouma T.J., Kleyer M. (2016) A global analysis of the seaward salt marsh extent: The importance of tidal range. Water Resources Research 52: 3775–3786.
Baptist M.J., Dankers P., Cleveringa J., Sittoni L., Willemsen P.W.J.M., van Puijenbroek M.E.B., de Vries B.M.L., Leuven J.R.F.W., Coumou L., Elschot K. (2021) Salt marsh construction as a nature-based solution in an estuarine social-ecological system. Nature Based Solutions 1: 100005.
Bartholdy A.T., Bartholdy J., Kroon A. (2010) Salt marsh stability and patterns of sedimentation across a backbarrier platform. Marine Geology 278: 31-42.
Bossinade, J.H., van den Bergs J., Dijkema K.S. (1993) De invloed van de wind op het jaargemiddelde hoogwater langs de Friese en Groninger waddenkust. Rijkswaterstaat Directie Groningen/DLO-Instituut voor Bos- en Natuuronderzoek. Texel. 22 pp.
Brooks K. L., Mossman, H. L., Chitty, J. L., Grant, A. (2015) Limited vegetation development on a created salt marsh associated with over-consolidated sediments and lack of topographic heterogeneity. Estuaries and Coasts. 38: 325-336.
Chang E., Veeneklaas, R.M., Bakker J.P., Esselink P. (2016) What factors determine restoration success of a salt marsh ten years after de-embankment? Applied vegetation science 19: 66-77.
Colina Alonso A., van Maren D.S., Oost A.P., Esselink P., Hagen R., Kösters F., Bartholdy J., Bijleveld A., Wang Z.B. (2024) A cross-border mud budget for future sediment management. Communications Earth & Environment 5: 153
Cornacchia L., Bakker, J.P., Koppenaal E.C., de Groot A.V. Olff H., van de Koppel, J., van der Wal, D., Bouma T.J. (2024) Spatial and temporal variation in long–term sediment accumulation in a back–barrier salt marsh. Geomorphology 456: 109191
Crosby S.C, Sax D.F., Palmer M.E., Booth H.S., Deegan L.A., Bertness M.D., Leslie H.M. (2016) Salt marsh persistence is threatened by predicted sea-level rise. Estuarine, Coastal and Shelf Science 181: 93–99.
CWSS (2010) Wadden Sea Plan 2010. Eleventh Trilateral Governmental Conference on the Protection of the Wadden Sea. Common Wadden Sea Secretariat, Wilhelmshaven. 102 pp.
de Groot A.V., Oost A.P., Veeneklaas R.M., Lammerts E.J., van Duin W.E., van Wesenbeeck B.K. (2016) Tales of island tails: biogeomorphic development and management of barrier islands. Journal of Coastal Conservation. p. 1–11.
de Vlas J., Mandema F.S., Nolte S., van Klink R., Esselink P. (2013) Nature conservation of salt marshes. Influence of grazing on biodiversity. PUCCIMAR-report 09, PUCCIMAR Ecological Research and Consultancy, Vries. It Fryske Gea, Olterterp. 32 pp.
Dijkema K.S. (1987a) Changes in salt marsh area in the Netherlands Wadden Sea after 1600. In: Huiskes A.H.L., Blom C.W.P.M., Rozema J. (eds). Vegetation between land and sea. Dr. W. Junk Publishers, Dordrecht. p. 42–49.
Dijkema K.S. (1987b) Geography of salt marshes in Europe. Zeitschrift für Geomorphologie 31: 489-499.
Dijkema K.S., Nicolai A., de Vlas J., Smit C.J., Jongerius H., Nauta H. (2001) Van landaanwinning naar kwelderwerken. Rijkswaterstaat Directie Noord-Nederland¸ Leeuwarden, Alterra, Texel. 68 pp.
Doody J.P. (2008) Saltmarsh conservation, management and restoration. Springer. 217 pp.
Drake B.G. (2014) Rising sea level, temperature, and precipitation impact plant and ecosystem responses to elevated CO2 on a Chesapeake Bay wetland: review of a 28-year study. Global Change Biology 20: 3329-3343.
Duarte C.M., Atwood T.B., Kairo J.G., Kennedy H., Krause-Jensen D., Lovelock C.E., Serrano O. (2021) UNESCO Marine World Heritage: custodians of the globe’s blue carbon assets. UNESCO, Paris, France.
Elschot K., Baptist M.J., van Puijenbroek M.E.B. (2023) Biocompacting livestock accelerate drowning of tidal salt marshes with sea level rise. Frontiers of Marine Science. 10: 1–19.
Elschot K., van Puijenbroek M.E.B., Lagendijk D.D.G., Van der Wal T.J., Sonneveld C. (2020) Lange-termijnontwikkeling van kwelders in de Waddenzee (1960-2018). Wettelijke Onderzoekstaken Natuur & Milieu, WOt-technical report 182, Wageningen Marine Research, Den Helder.
Esselink P. (1998) Van landaanwinning naar natuurbeheer: Recente ontwikkelingen op de Dollardkwelders. In: Essink K., Esselink P. (eds). Het Eems-Dollard estuarium: interacties tussen menselijke beïnvloeding en natuurlijke dynamiek. Rapport RIKZ-98-020. Rijkswaterstaat Rijksinstituut voor Kust en Zee / RIKZ, Haren. p. 79–99.
Esselink P. (2000) Nature management of coastal salt marshes. Interactions between anthropogenic influences and natural dynamics. PhD-thesis. University of Groningen, Groningen. 256 pp.
Esselink P., Bos D., Daniels P., van Duin W.E., Veeneklaas R.M. (2015) Van polder naar kwelder: tien jaar kwelderherstel Noarderleech. PUCCIMAR-rapport 06, PUCCIMAR Ecologisch Onderzoek & Advies, Vries. 209 pp.
Esselink P., Petersen J., Arens S., Bakker J.P., Bunje J., Dijkema K.S., Hecker N., Hellwig U., Jensen A.-V., Kers A.S., Körber P., Lammerts E.J., Stock M., Veeneklaas R.M., Vreeken M., Wolters M. (2009) Salt Marshes. Thematic Report No. 8. In: H. Marencic & J. de Vlas (eds). Quality Status Report 2009. Wadden Sea Ecosystem No. 25. Common Wadden Sea Secretariat, Trilateral Monitoring and Assessment Group, Wilhelmshaven.
Esselink, P., van Duin, W. E., Bunje, J., Cremer, J., Folmer, E., Frikke, J., Glahn, M., de Groot, A. V., Hecker, N., Hellwig, U., Jensen, K., Körber, P., Petersen, J., & Stock, M. (2017). Wadden Sea Quality Status Report: Salt marshes. Common Wadden Sea Secretariat. https://doi.org/10.5281/zenodo.15196554
French J.R. (1993) Numerical-Simulation of Vertical Marsh Growth and Adjustment to Accelerated Sea-Level Rise, North Norfolk, UK. Earth Surface Processes and Landforms. 18(1): 63–81.
Granse D., Suchrow S., Jensen K. (2021) Long-term invasion dynamics of Spartina increase vegetation diversity and geomorphological resistance of marshes against sea level rise. Biological Invasions. 23: 871-883.
Govers L., Reijers V., Smeele Q., Olff H., Temmink R., Marin-Diaz B., Fivash G., Nauta J., van der Heide T. (2021) Habitat-overstijgende interacties in het Waddengebied. Rap.nr. 2021/OBN248-DK, VBNE, Driebergen.
Hartmann K., Stock M. (2019) Long-term change in habitat and vegetation in an ungrazed, estuarine salt marsh: Man-made foreland compared to young marsh development. Estuarine, Coastal and Shelf Science 227: 106348.
Hofstede J., Schirmacher R. (1996) Vorlandmanagement in Schleswig-Holstein. Die Küste 58: 61-73
Jensen J., Dangendorf S., Wahl T., Steffen H. (2014) Meeresspiegeländerungen in der Nordsee: Vergangene Entwicklungen und zukünftige Herausforderungen mit einem Fokus auf die Deutsche Bucht. Hydrologie und Wasserbewirtschaftung 58: 304–323.
Kleefstra R., Bregnballe T., Frikke J., Günther K., Hälterlein B., Hansen M.B., Hornman M., Ludwig, J., Meyer, J., Scheiffarth G. (2022) Trends of Migratory and Wintering Waterbirds in the Wadden Sea 1987/1988 - 2019/2020. Wadden Sea Ecosystem No. 41. Common Wadden Sea Secretariat, Expert Group Migratory Birds, Wilhelmshaven, Germany.
Koppenaal E.C., Esselink P., van Duin W.E., Bakker J.P. (2022) Temporal and spatial accretion patterns and the impact of livestock grazing in a restored coastal salt marsh. Estuaries and Coasts. 45: 510-522.
Kirwan M.L., Guntenspergen G.R., D’Alpaos A., Morris J.T., Mudd S.M., Temmerman S. (2010) Limits on the adaptability of coastal marshes to rising sea level. Geophysical Research Letter 37:L23401.
Kirwan M.L., Temmerman S., Skeehan E.E., Guntenspergen G.R., Fagherazzi S. (2016) Overestimation of marsh vulnerability to sea level rise. Nature Climate Change 6: 253–260.
Mueller P., Ladiges N., Jack A., Schmiedl G., Kutzbach L., Jensen K., Nolte S. (2019) Assessing the long-term carbon-sequestration potential of the semi-natural salt marshes in the European Wadden Sea. Ecosphere. 10(1): e02556.
Nationalparkverwaltung Niedersächsisches Wattenmeer (2022) Managementmaßnahmen im Nationalpark “Niedersächsisches Wattenmeer“ mit Darstellung der Erhaltungsmaßnahmen im gleichnamigen FFH-Gebiet 001. Anlage 1.7: Maßnahmenblatt LRT 1330 Atlantische Salzwiesen. https://www.nationalpark-wattenmeer.de/wissensbeitrag/ffh-massnahmenpla…
Nehring S., Hesse K.J. (2008) Invasive alien plants in marine protected areas: the Spartina anglica affair in the European Wadden Sea. Biological invasions. 10: 937-950.
Nolte S. (2014) Grazing as a nature management tool. The effect of different livestock species and stocking densities on salt-marsh vegetation and accretion. PhD-thesis. University of Groningen, Groningen. 245 pp.
Nolte S., Koppenaal E.C., Esselink P., Dijkema K.S., Schuerch M., de Groot A.V., Bakker J. P., Temmerman S. (2013) Measuring sedimentation in tidal marshes: a review on methods and their applicability in biogeomorphological studies. Journal of Coastal Conservation. 17: 301–325.
Odum W.E. (1988) Comparative ecology of tidal freshwater and salt marshes. Annual Review of Ecology and Systematics 19: 147–176.
Olff H., Leeuw, J. De, Bakker, J.P., Platerink, R.J., van Wijnen, H.J. (1997) Vegetation Succession and Herbivory in a Salt Marsh: Changes Induced by Sea Level Rise and Silt Deposition Along an Elevational Gradient. Journal of Ecology. 85: 799. doi: 10.2307/2960603.
Oost A.P., Colina Alonso A., Esselink P., Wang Z.B., van Kessel T., van Maren B. (2021) Where mud matters. Towards a mud balance for the trilateral Wadden Sea Area: Mud supply, transport and deposition. Wadden Akademie, Rapport 2021-02.
Oost, A. P., Winter, C., Vos, P., Bungenstock, F., Schrijvershof, R., Röbke, B., Bartholdy, J., Hofstede, J., Wurpts, A., & Wehrmann, A. (2017). Wadden Sea Quality Status Report: Geomorphology. Common Wadden Sea Secretariat. https://doi.org/10.5281/zenodo.15102363
Petersen J., Kers B., Stock M. (2014) TMAP-typology of coastal vegetation in the Wadden Sea area. Wadden Sea Ecosystems 32: 1–86. Common Wadden Sea Secretariat, Wilhelmshaven.
Philippart, C., Baptist, M., Bastmeijer, K., Bregnballe, T., Buschbaum, C., Hoekstra, P., Laursen, K., van Leeuwen, S. M., Oost, A. P., Wegner, M., & Zijlstra, R. (2024). Wadden Sea Quality Status Report: Climate Change. Common Wadden Sea Secretariat. https://doi.org/10.5281/zenodo.15111640
Reise K. (1994) Das Schlickgras Spartina anglica: die Invasion einer neue Art. In: J.L. Lozán, E. Rachor, K. Reise, H. von Westernhagen & W. Lenz (eds). Warnsignale aus dem Wattenmeer. Blackwell Wissenschafts-Verlag, Berlin. p. 211–214.
Rupprecht F., Reichert G., Merling B., Oltmanns B. (2023) Renaturierung von Salzwiesen im Nationalpark Niedersächsisches Wattenmeer. Berichte aus dem Nationalpark und der Biospärenregion, Niedersächsisches Wattenmeer 2023-03.
Rupprecht F., Wanner A., Stock M., Jensen K. (2015) Succession in salt marshes – large-scale and long-term patterns after abandonment of grazing and drainage. Applied Vegetation Science. 18: 86–98.
Schindler M., Karius V., Arns A., Deicke M., von Eynatten H. (2014) Measuring sediment deposition and accretion on anthropogenic marshland - Part II: The adaptation capacity of the North Frisian Halligen to sea level rise. Estuarine, Coastal and Shelf Science 151: 246-255.
Schuerch M., Spencer T., Temmerman S., Kirwan M.L., Wolff C., Lincke D., McOwen C.J., Pickering M.D., Reef R., Vafeidis A.T., Hinkel J., Nicholls R.J., Brown S. (2018) Future response of global wetlands to sea-level rise. Nature 561: 231-234.
Schulze D., Jensen K., Nolte, S. (2021) Livestock grazing reduces sediment deposition and accretion rates on a highly anthropogenically altered marsh island in the Wadden Sea. Estuarine, Coastal and Shelf Science. 251: 107191.
Stock M. (1997) Salt marsh management in the National Park "Schleswig-Holsteinisches Wattenmeer". Wadden Sea Newsletter 1997(1): 11–15.
Stock M., Maier M. (2016) Salzwiesenschutz im Nationalpark Wattenmeer – ein Überblick. Vogelkundliche Berichte aus Niedersachsen 44: 131-156.
Temmerman S., Meire P., Bouma T.J., Herman P.M.J., Ysebaert T., De Vriend H.J. (2013) Ecosystem-based coastal defence in the face of global change. Nature 504: 79-83.
van Duin W.E., Dijkema K.S. (2003) Proef met de onderhoudsarme ontwatering in de kwelderwerken: ”de Krekenproef”; evaluatie 1997-2002. Alterra-rapport 634, Alterra, Wageningen.
van Eerde L.A.E. (1942) De landaanwinning van het Noorderleegs Buitenveld. Tijdschrift Nederlands Aardrijkskundig Genootschap 59: 1–23.
van Klink R. (2014) Of dwarves and giants – how large herbivores shape arthropod communities on salt marshes. PhD-thesis. University of Groningen, Groningen. 227 pp.
van Klink R., Nolte S., Mandema F.S., Lagendijk D.D.G., WallisdeVries M.F., Bakker J.P., Esselink P., Smit C. (2016) Effects of grazing management on biodiversity across trophic levels – the importance of livestock species and stocking density in salt marshes. Agriculture, Ecosystems and Environment 235: 329–329.
van Puijenbroek M.E.B., van den Dool R.T., Koppenaal E.C., Smit C., Berende F., Limpens J., Bakker J.P. (2021) Green beach vegetation dynamics explained by embryo dune development. Basic and Applied Ecology 56: 45-57.
van Wesenbeeck B.K., Esselink P., Oost A.P., van Duin W.E., de Groot A.V., Veeneklaas R.M., Balke T., van Geer P., Calderon A.C., Smale A. (2014) Verjonging van half-natuurlijke kwelders en schorren. Rapport nr. 2014/OBN196-DK. VBNE, Vereniging van Bos- en Natuurterreineigenaren, Driebergen. 73 pp.
Vos P.C., Knol E. (2015) Holocene landscape reconstruction of the Wadden Sea area between Marsdiep and Weser. Netherlands Journal of Geosciences 94: 157–183.
Wahl T., Jensen J., Frank T., Haigh I. (2011) Improved estimates of mean sea level changes in the German Bight over the last 166 years. Ocean Dynamics 61: 701–715.
Wanner A., Suchrow S., Kiehl K., Meyer W., Pohlmann N., Stock M., Jensen K. (2014) Scale matters: Impact of management regime on plant species richness and vegetation type diversity in Wadden Sea salt marshes. Agriculture, Ecosystems and Environment 182: 69–79.
Wiersma A.P., Oost A.P., van der Berg M.W., Vos P.C., Marges V., de Vries S. (2009) Geomorphology. Thematic Report No. 9. In: Marencic H., de Vlas J. (eds). 2009. Quality Status Report 2009. Wadden Sea Ecosystem No. 25. Common Wadden Sea Secretariat, Wilhelmshaven.
Wolters M., Garbutt A., Bakker J.P. (2005) Salt-marsh restoration: evaluating the success of de-embankments in north-west Europe. Biological Conservation 123: 249–268.
The content of the report is the sole responsibility of the author(s) and does not reflect the position of the Trilateral Governmental Cooperation for the Protection of the Wadden Sea (TWSC), its member organisations, or the Common Wadden Sea Secretariat (CWSS).
This report should be cited as: Elschot, K., Esselink, P., Frikke, J., Jensen, K., Janinhoff-Verdaat, N., Paarup Thomsen, M., Padlat, M., van der Wal, J. T., van Duin, W. E., van Puijenbroek, M., Rupprecht, F., & Stock, M. (2024). Wadden Sea Quality Status Report: Salt marshes. Common Wadden Sea Secretariat. https://doi.org/10.5281/zenodo.15196067
All 2024 reports may be cited collectively as: Kloepper, S., Bostelmann, A., Bregnballe, T., Busch, J.A., Buschbaum, C., Deen, K., Domnick, A., Gutow, L., Jensen, K., Jepsen, N., Luna, S., Meise, K., Teilmann, J., & van Wezel, A. (2024). Wadden Sea Quality Status Report. Common Wadden Sea Secretariat, Wilhelmshaven, Germany. Downloaded DD.MM.YYYY. qsr.waddensea-worldheritage.org